How Well are You Aging? BDNF, TREM2 & Telomere Length as Biomarkers of Aging
Docere
Angela Knapp, ND
Since the inception of the human genome project in the 1990s, we humans have been looking to our DNA for the promotion of health and longevity.1 For the past 2 decades, the research, knowledge, and understanding of the field of genetics have grown exponentially, and we are finally beginning to gain an appreciation of how genetics may allow us to bio-hack our health and lengthen human lifespan. In the past 5 years, in particular, we have increased our understanding of a wide range of single nucleotide polymorphisms that may promise a greater insight into how our bodies and brains are aging. Telomeres, a different aspect of our DNA, have been under the spotlight due to the recent research associating telomere length with aging, cancer development, and inflammation. Since both genetics and telomere length influence our rate of aging, measuring them may move us closer to attaining that elusive fountain of youth by highlighting affected pathways that can be modified.
Single nucleotide polymorphisms (SNPs) are the base-pair changes in amino acid sequences within our genes that make one person different from another. A significant number of SNPs have been associated with alterations in enzyme function, protein structure, and pathology.2 Among the best-known SNPs are APOE and MTHFR. In an effort to move beyond the buzz words, this particular article discusses SNPs that have been the focus of prominent research within the past 5 years, such as BDNF and TREM2. Although the quantity of research on these SNPs is less extensive compared to some others, it is significant and rapidly expanding.
BDNF
Brain-derived neurotrophic factor (BDNF) was isolated from pig brain in 1989 by Yves-Alain Barde and Hans Thoenen.3 This discovery opened the door to research on BDNF in humans. BDNF is a gene that encodes for the BDNF protein, which is part of the neurotrophin family. Neurotrophins are cell-signaling molecules that have the ability to promote survival, development, and function of neurons while also preventing the initiation of programmed cell death.3 Neurotrophins are integral to the nervous system’s development and plasticity.4 This signaling molecule therefore plays a pertinent role in brain and nervous system function. Research has found that BDNF has neuroprotective effects, contributes to neurogenesis, and modifies aspects of synaptic plasticity.
The Val66Met polymorphism in the rs6265 gene that codes for BDNF has been associated with compromising effects to the structure of the brain, such as modified synaptic and cellular plasticity.5 A 2003 study showed that the Met allele is associated with reduced recall of episodic memory, as compared with the common Val allele.6 Furthermore, 2 MRI investigations found that carriers of the Met allele showed reduced hippocampal volumes and reduced prefrontal cortex gray matter, as compared to Val homozygotes.5,7
It is well recognized that hippocampal atrophy is associated with aging. So, what can one do when he or she has a Met allele, cognitive decline, and fear of aging? This is where the beauty of epigenetics comes in. Research on BDNF levels has found that regardless of carriage of the Met allele, high-intensity exercise not only increases serum BDNF levels, but also non-significantly improves learning of a novel locomotor pattern (previous studies suggested a greater impact).8 Interestingly, multiple in-vivo and in-vitro studies have found botanicals such as Bacopa monnieri, Coffea arabica, and Panax ginseng to attenuate the negative affects of a Met allele and the cellular damage associated with reduced BDNF mRNA levels (Figure 1).9
Figure 1. Botanical Effects on BDNF
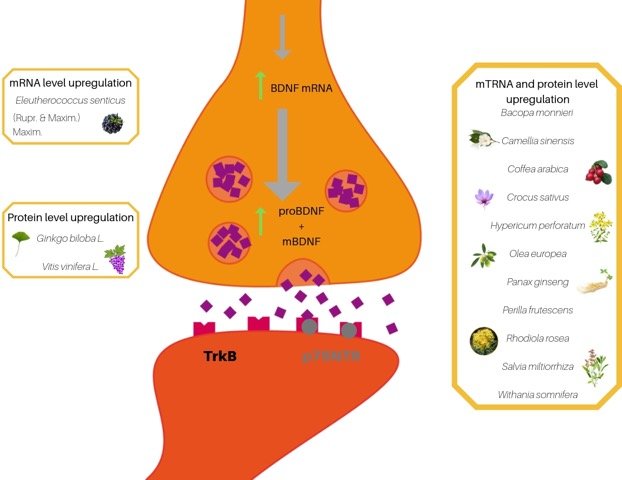
(Image adapted from Sangiovanni et al. Botanicals as Modulators of Neuroplasticity: Focus on BDNF. Neural Plast. 2017;2017:5965371.)
The figure illustrates botanicals acting at translational and transcriptional levels.
TREM
TREM is an abbreviation for the protein-triggering receptor expressed on myeloid cells. There are both TREM1 and TREM2 proteins. TREM1 is known to be proinflammatory, while TREM2 is associated with reduced production of proinflammatory cytokines, ie, is anti-inflammatory. TREM proteins are expressed on monocytes, macrophages, and microglial cells.10 TREM2 has been shown to be an immunomodulatory receptor, and a TREM2 genetic variant (rs75932628) has been associated with increased risk of Alzheimer’s disease and neurodegenerative disorders.11,12
TREM2 variants are rare in the population but have been associated with a risk as significant as APOE4 for late-onset Alzheimer’s disease as well as other neurodegenerative diseases, such as Parkinson’s disease and sporadic amyotrophic lateral sclerosis (ALS).13-16 The mechanism of TREM2 and amyloid plaque formation has been postulated, but is yet to be determined.11 Multiple studies have pointed to TREM2 as a protein that supports anti-inflammatory pathways, yet alternative data suggests that the role of TREM2 may be significantly more complicated.17 The complexity and lack of clarity as to the exact mechanism behind TREM2’s relationship to neurodegenerative disorders continues to ongoing investigations. In the meantime, should you identify this rare variant in your patients, interventions aimed at immunomodulation and reducing neuroinflammation should be emphasized. Significant evidence has also shown that exercise, as discussed above for BDNF, can support cognitive function and reduce neurodegeneration, in general.
Telomeres
Telomeres are a different aspect of DNA than a SNP. Telomeres are considered to be the protective tail (or cap) of chromosomes, creating a barrier for the DNA required to code for life-dependent proteins. Telomeres are non-coding DNA. Each chromosome is composed of a repetitive sequence of 6 proteins that make up the telomere. This 6-protein complex is termed “shelterin” because its role is predominantly to conceal the telomeric DNA and protect it from harm.18 However, with each cell division, a telomere is shortened, which is why telomeres have been studied for their association with aging and even life expectancy.19
The telomere theory of aging considers the shortening of telomeres to be the cause of the aging process. This conflicts with the theory of oxidative stress as the source of aging. Although there is research correlating average telomere length with mortality, the research is still highly contradictory.20 Research has revealed that telomere length is variable depending on the tissue type. For example, length may tend to be greater in women than in men.21 Telomere length can be dynamically shifted based on one’s exposure to stress and environmental factors.22-24 Nevertheless, because neurodegenerative disorders have been associated with premature cell death, and telomere length is an indicator of cellular senescence, the length of telomeres may play an important role in monitoring progression of disease. Research on exercise and telomere length has revealed a clear association between increased telomere length and high levels of physical activity.22 Lifestyle factors and nutritional supplementation may also affect telomere length. According to the evidence, telomeres can be lengthened by social support, meditation, reduced stress, high-quality sleep, omega-3 fatty acids, vitamin D (at least in women), and green tea.19,25-29
Summary
This article just begins to scratch the surface of the potential that telomere measurement and genetic testing has to offer for maximizing brain health and reducing aging. Any good clinician, however, will look at how a lab test influences a treatment plan. How do we use these multiple genetic markers in our treatment of patients? While BDNF and TREM2 polymorphisms may indicate increased risks, the lifestyle factors utilized to manage these risks are generally ones we already discuss with our patients. What these markers can do is encourage us to emphasize the importance and intensity of our treatment modalities for relevant patients. The same holds for telomere length when we discuss lifestyle with our patients. As the research associated with these genetic markers continues to evolve, we may be able to better direct our application of modalities at the precise mechanisms affected by these pathways and biological processes, such as the botanicals reviewed in the article by Sangiovanni et al.9 In the meantime, the research does underline the importance of preventative medicine and lifestyle as the key to health.
References:
- Humphreys KL, Esteves K, Zeanah CH, et al. Accelerated telomere shortening: Tracking the lasting impact of early institutional care at the cellular level. Psychiatry Res. 2016;246:95-100.
- Moraes F, Góes A. A decade of human genome project conclusion: Scientific diffusion about our genome knowledge. Biochem Mol Biol Edu. 2016;44(3):215-223.
- Kowiański P, Lietzau G, Czuba E, et al. BDNF: A Key Factor with Multipotent Impact on Brain Signaling and Synaptic Plasticity. Cell Mol Neurobiol. 2018:38(3):579-593.
- Baj G, Carlin D, Gardossi L, Tongiorgi E. Toward a unified biological hypothesis for the BDNF Val66Met-associated memory deficits in humans: a model of impaired dendritic mRNA trafficking. Front Neurosci. 2013:7:188.
- Pezawas L, Verchinski BA, Mattay VS, et al. The brain-derived neurotrophic factor val66met polymorphism and variation in human cortical morphology. J Neurosci. 2004:24(45):10099-10102.
- Egan MF, Kojima M, Callicott JH, et al. The BDNF val66met polymorphism affects activity-dependent secretion of BDNF and human memory and hippocampal function. Cell. 2003:112(2):257-269.
- Szeszko PR, Lipsky R, Mentschel C, et al. Brain-derived neurotrophic factor val66met polymorphism and volume of the hippocampal formation. Mol Psychiatry. 2005:10(7):631-636.
- Helm EE, Matt KS, Kirschner KF, et al. The influence of high intensity exercise and the Val66Met polymorphism on circulating BDNF and locomotor learning. Neurobiol Learn Mem. 2017:144:77-85.
- Sangiovanni E, Brivio P, Dell’Agli M, Calabrese F. Botanicals as Modulators of Neuroplasticity: Focus on BDNF. Neural Plast. 2017;2017:5965371.
- Subramanian S, Pallati P, Rai V, et al. Increased expression of triggering receptor expressed on myeloid cells-1 in the population with obesity and insulin resistance. Obesity (Silver Spring). 2017:27(3):513-515.
- Kober D, Brett T. TREM2-Ligand Interactions in Health and Disease. J Mol Biol. 2017:429(11):1607-1629.
- Guerreiro R, Wojtas A, Bras J, et al. TREM2 variants in Alzheimer’s disease. N Engl J Med. 2013:368(2):117-127.
- Golde TE, Streit WJ, Chakrabarty P. Alzheimer’s disease risk alleles in TREM2 illuminate innate immunity in Alzheimer’s disease. Alzheimers Res Ther. 2013;5(3):24.
- Rayaprolu S, Mullen B, Baker M, et al. TREM2 in neurodegeneration: evidence for association of the p.R47H variant with frontotemporal dementia and Parkinson’s disease. Mol Neurodegener. 2013;8:19.
- Liu G, Liu Y, Jiang Q, et al. Convergent Genetic and Expression Datasets Highlight TREM2 in Parkinson’s Disease Susceptibility. Mol Neurobiol. 2016;53(7):4931-4938.
- Cady J, Koval ED, Benitez BA, et al. TREM2 variant p.R47H as a risk factor for sporadic amyotrophic lateral sclerosis. JAMA Neurol. 2014;71(4):449-453.
- Jay TR, von Saucken VE, Landreth GE. TREM2 in Neurodegenerative Diseases. Mol Neurodegener. 2017;12(1):56.
- Aubert G. Telomere Dynamics and Aging. In: Calado RT, ed. Progress in Molecular Biology and Translational Science. Volume 25: Telomeres in Health and Disease. Waltham, MA: Elsevier; 2014:89-111.
- Chopra D, Tanzi R. The Telomere Connection. Alternative and Complementary Therapies. 2018;24(4):167-169.
- Bernadotte, Mikhelson VM, Spivak IM. Markers of cellular senescence. Telomere shortening as a marker of cellular senescence. Aging (Albany NY). 2016;8(1):3-11.
- Dalgård C, Benetos A, Verhulst S, et al. Leukocyte telomere length dynamics in women and men: menopause vs age effects. Int J Epidemiol. 2015;44(5):1688-1695.
- Arsenis NC, You T, Ogawa EF, et al. Physical activity and telomere length: Impact of aging and potential mechanisms of action. Oncotarget. 2017;8(27):45008-45019.
- Shammas MA. Telomeres, lifestyle, cancer, and aging. Curr Opin Clin Nutr Metab Care. 2011;14(1):28-34.
- Yang CW, Tseng SF, Yu CJ, et al. Telomere shortening triggers a feedback loop to enhance end protection. Nucleic Acids Res. 2017;45(14):8314-8328.
- Rathore M, Abraham J. Implication of Asana, Pranayama and Meditation on Telomere Stability. Int J Yoga. 2018;11(3):186-193.
- Epel E, Daubenmier J, Moskowitz JT, et al. Can meditation slow rate of cellular aging? Cognitive stress, mindfulness, and telomeres. Ann N Y Acad Sci. 2009;1172:34-53.
- Farzaneh-Far R, Lin J, Epel ES, et al. Association of marine omega-3 fatty acid levels with telomeric aging in patients with coronary heart disease. JAMA. 2010;303(3):250-257.
- Richards JB, Valdes AM, Gardner JP, et al. Higher serum vitamin D concentrations are associated with longer leukocyte telomere length in women. Am J Clin Nutr. 2007;86(5):1420-1425.
- Chan R, Woo J, Suen E. Chinese tea consumption is associated with longer telomere length in elderly Chinese men. Br J Nutr. 2010;103(1):107-113.
Angela Knapp, ND, is a 2013 graduate of the National University of Natural Medicine (NUNM). Dr Knapp focuses her practice on pediatric chronic illness and hosts a Facebook Live series on PANS and PANDAS. She is the staff physician for Kashi Clinical Laboratories, where she provides research and development on genetic testing and counsels physicians on genetic testing results. She is the proud mother of two wonderful, dynamic children.