Adrenal Fatigue: Environmentally Induced Adrenal Hypofunction?
Tolle Causam
Andrea Gruszecki, ND
Normal adrenal gland function is essential during acute and chronic stress responses.1 Although commonly used, the term “adrenal fatigue” is not considered a diagnosable illness by allopathic medicine, perhaps because the effects of bioaccumulated background toxic exposures are not usually considered by allopathic physicians.2,3 The available evidence indicates that adrenal insufficiency may occur from a variety of factors, including accumulated toxic exposures.4 However, the testing guidelines currently used to assess endocrine disruption have neglected the examination of the adrenal gland, and have not fully explored chemical effects on steroid hormone receptors and metabolism.5 It is especially important, then, to consider the effects of toxic exposures on adrenal function. Toxic exposures increase oxidative stress and alter enzyme activity.3-6 These effects, combined with poor nutrition and inherited variations in enzymes of steroid hormone metabolism and detoxification, may contribute to adrenal hypofunction in susceptible individuals.4 Relieving toxic burden may help prevent the progression to autoimmune adrenal insufficiency and improve adrenal function in those at risk for environmentally induced adrenal hypofunction.7,8
Adrenal Hypofunction
With our increasing understanding of individual genetic variations in enzyme function and detoxification capacity, it is likely that what has been termed “adrenal fatigue” in the past actually describes an environmentally induced adrenal hypofunction. Chronic stress is also part of one’s environment and is known to alter cortisol levels, particularly if the stressful condition cannot be avoided or changed.9 Toxic exposures may affect the hypothalamus-pituitary-adrenal (HPA) axis in several different ways. The exposure may alter glucocorticoid or mineralocorticoid metabolism, central nervous system signaling of the HPA axis, or the expression of receptors. Changes that result from toxic exposures or chronic stress may be reflected in abnormal cortisol slopes (Figure 1) and altered urinary glucocorticoid and mineralocorticoid metabolite levels.4,10,11
Figure 1. Flattened Cortisol Curve
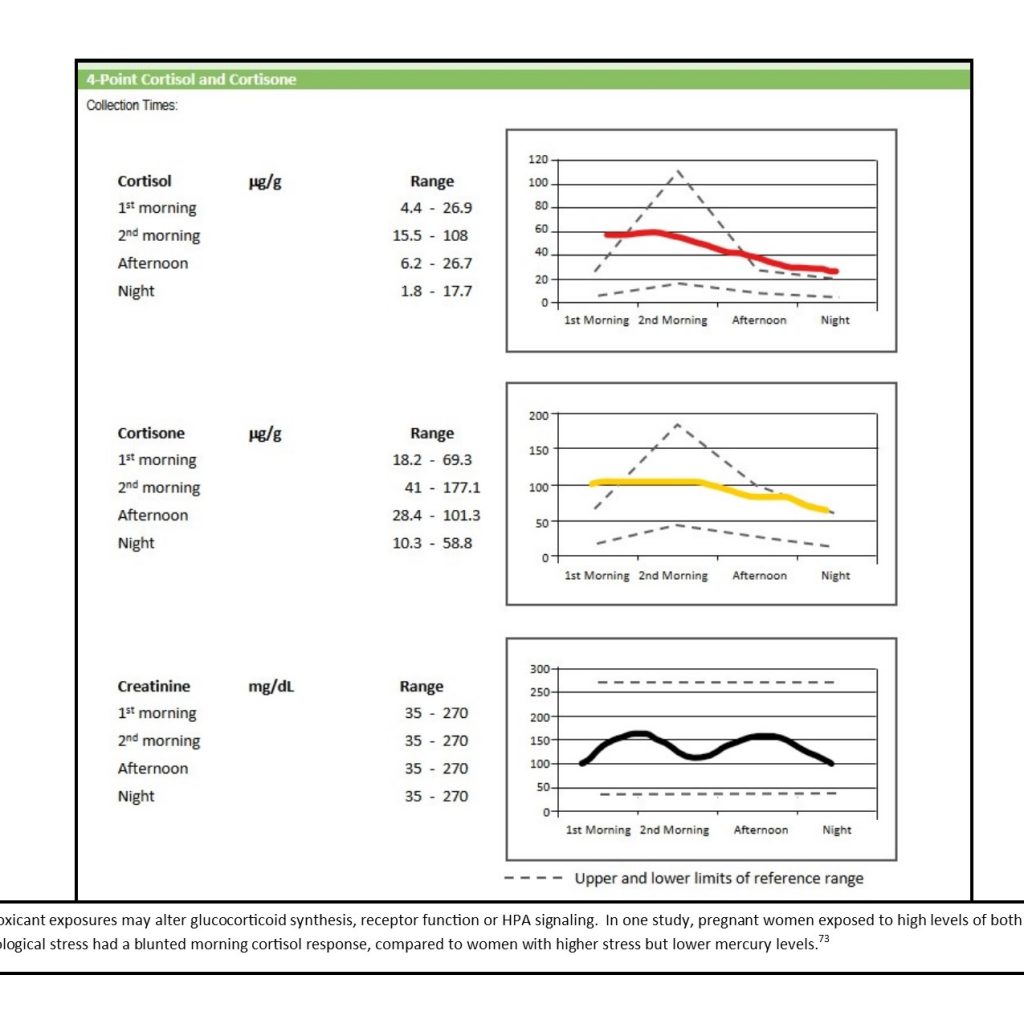
However, before adrenal hypofunction can be blamed wholly on chronic psychological stress or environmental exposures, inherited and physiological causes of adrenal hypofunction must be ruled out.2 Symptoms of adrenal insufficiency include fatigue, anorexia, and weight loss; other symptoms, such as nausea, hyperpigmentation, salt craving, hypotension, abdominal pain, altered gastrointestinal (GI) motility, and postural dizziness, may also be present. Organic causes include the following:
- Primary adrenal insufficiency: This occurs when adrenal glands are underactive due to damage by organ-specific antibodies or genetics.13-15 Up to 90% of recent-onset primary adrenal insufficiency cases present with 21-hydroxylase antibodies and other anti-adrenal antibodies that damage the adrenal gland. It has been shown that up to 75% of individuals with Addison’s disease have such autoimmune damage; however, symptoms of insufficiency may not become apparent until more than 80% of the adrenal cortex has been compromised.16,17 Up to 50% of individuals diagnosed with Addison’s may have, or may develop, a second autoimmune condition.
- Secondary adrenal insufficiency: This may occur due to the sudden discontinuation of glucocorticoid medications, use of oral contraceptives, hypothyroid dysfunction, or pituitary disorders that decrease adrenocorticotropic hormone (ACTH).18
- Tertiary adrenal insufficiency: This results from hypothalamus disorders or an insufficient secretion of corticotropin-releasing hormone (CRH).
- Congenital adrenal hyperplasia (CAH): This is due to genetic mutations in the enzymes on the steroidal sex-hormone synthesis pathway, usually 21-hydroxylase (aka CYP21A2; heme cofactor) or 11β-hydroxysteroid dehydrogenase type 3 (HSD3B1; B3 cofactor). Most patients with CAH are diagnosed in infancy and present with abnormal genitalia; however, occasional atypical presentations are not diagnosed until puberty or adulthood.19 Other inherited low-activity variations in these enzymes, or in steroid hormone metabolic or detoxification enzymes, may also increase an individual’s susceptibility to the effects of toxic exposures.20
- Comorbid conditions such as chronic stress (eg, adverse childhood events, low socioeconomic status, posttraumatic stress disorder, or menstrual or menopausal mood disorders), chronic fatigue syndrome, rheumatoid arthritis, asthma, eczema, chronic pelvic pain, irritable bowel syndrome, etc, have been associated with adrenal hypofunction.9,21,22
Adrenal Effects of Toxic Exposures
It is also possible that environmental exposures play a role in the induction of autoimmune primary adrenal insufficiency (ie, Addison’s disease). Antibody screening may be used during the diagnostic evaluation of individuals with Addison’s or be used to monitor asymptomatic at-risk individuals (family history, high-risk genetics, etc).14 Fortunately, the presence of 21-hydroxylase antibodies does not mandate progression to Addison’s, as only 30% of those with positive antibodies typically progress to active disease.14 The low percentage of disease progression provides another clue that environmental factors may play a larger role than expected, and it is possible that early-stage, subclinical, antibody-positive individuals might best benefit from detoxification strategies.7,23 Toxic chemical exposures are known to induce autoimmune responses, and while Addison’s disease is considered irreversible, there are several documented cases of spontaneous remission.24,25 Such cases may be another clue that environmental conditions, including toxic exposures from metals or persistent organic pollutants (polychlorinated biphenyls, organochlorines, benzenes, etc) may play a role in disease induction and recovery.26,27 In addition, toxicants may directly damage mitochondria, which contain several enzymes vital for steroidogenesis, and toxicants may alter liver detoxification functions, which can affect circulating glucocorticoid and sex hormone levels.28,29
Enzymes
Toxic exposures can compromise glucocorticoid synthesis and metabolism; each enzyme in the glucocorticoid synthesis pathway can be affected by multiple toxicants.3,4,30,31 Over 60 chemicals are known to alter adrenocortical functions, and perinatal exposures or exposures during developmental windows may alter the function of the HPA axis for life.30-33 The adrenal gland and cortex have been documented to have the greatest sensitivity to toxicants of all the endocrine organs.30 Nutrient deficiencies can exacerbate the effects of toxic exposures, so the cofactor requirements of the pathway enzymes need to be considered as well. Cortisol is synthesized in the adrenal glands by cytochrome (CYP) P450 enzymes, which require a heme cofactor. Heme synthesis may be compromised as a result of iron deficiency, toxic metal exposures, or the presence of porphyria.3,34 Cortisol converts into its storage form, cortisone, via 11β-hydroxysteroid dehydrogenase type 2 (HSD11B2; B3 cofactor).35 Cortisone may be converted into cortisol as needed by 11β-hydroxysteroid dehydrogenase type 1 (HSD11B1; B3 cofactor), a different enzyme. The ratio between urinary free cortisol and cortisone, along with the ratio of cortisol metabolites to cortisone metabolites, indicates the activity of HSD11B1 and HSD11B2 (Figure 2).
Figure 2. Altered Glucocorticoid Levels
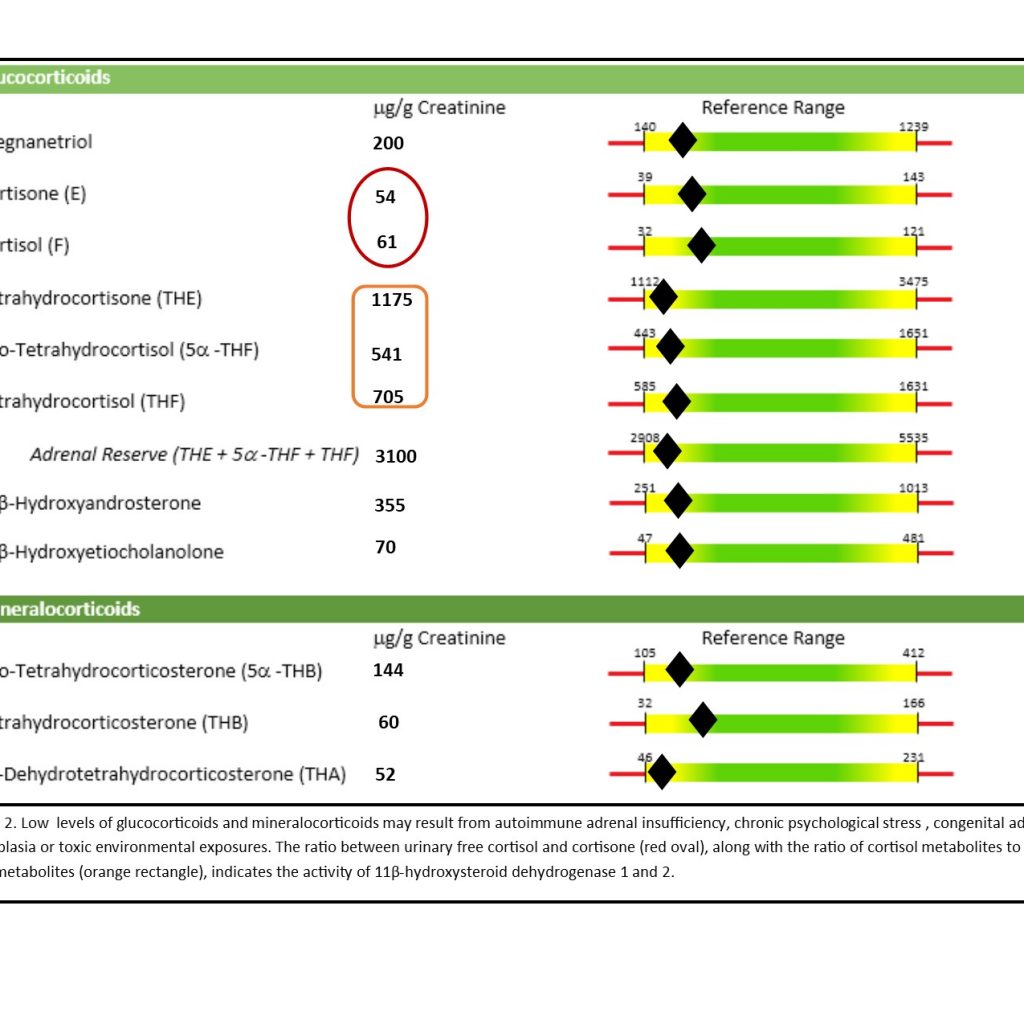
HSD11B1 activity requires a functional hexose-6-phosphate dehydrogenase pathway and nicotinamide adenine dinucleotide (NAD+) availability. The organic acids, kynurenic acid and xanthurenic acid, may provide clues regarding NAD+ status (Figure 3).36,37 Cell studies indicate that toxic metals such as cadmium, cobalt, copper, and mercury can decrease adrenal cell viability and cortisone, while animal studies suggest that mercury and cadmium exposures can decrease corticosterone levels and induce adrenal hyperplasia.3 The adreno-toxic effects of chemicals and metabolites from the polychlorinated biphenyls (PCBs) and dichloro-diphenyl-trichloroethane (DDT) family (mitotane, etc) may include altered function of steroidogenic enzymes such as mitochondrial CYP450 enzymes (CYP11A1, CYP11B1; heme cofactor), and reduce the levels of glucocorticoids.4
Figure 3. Kynurenic Acid
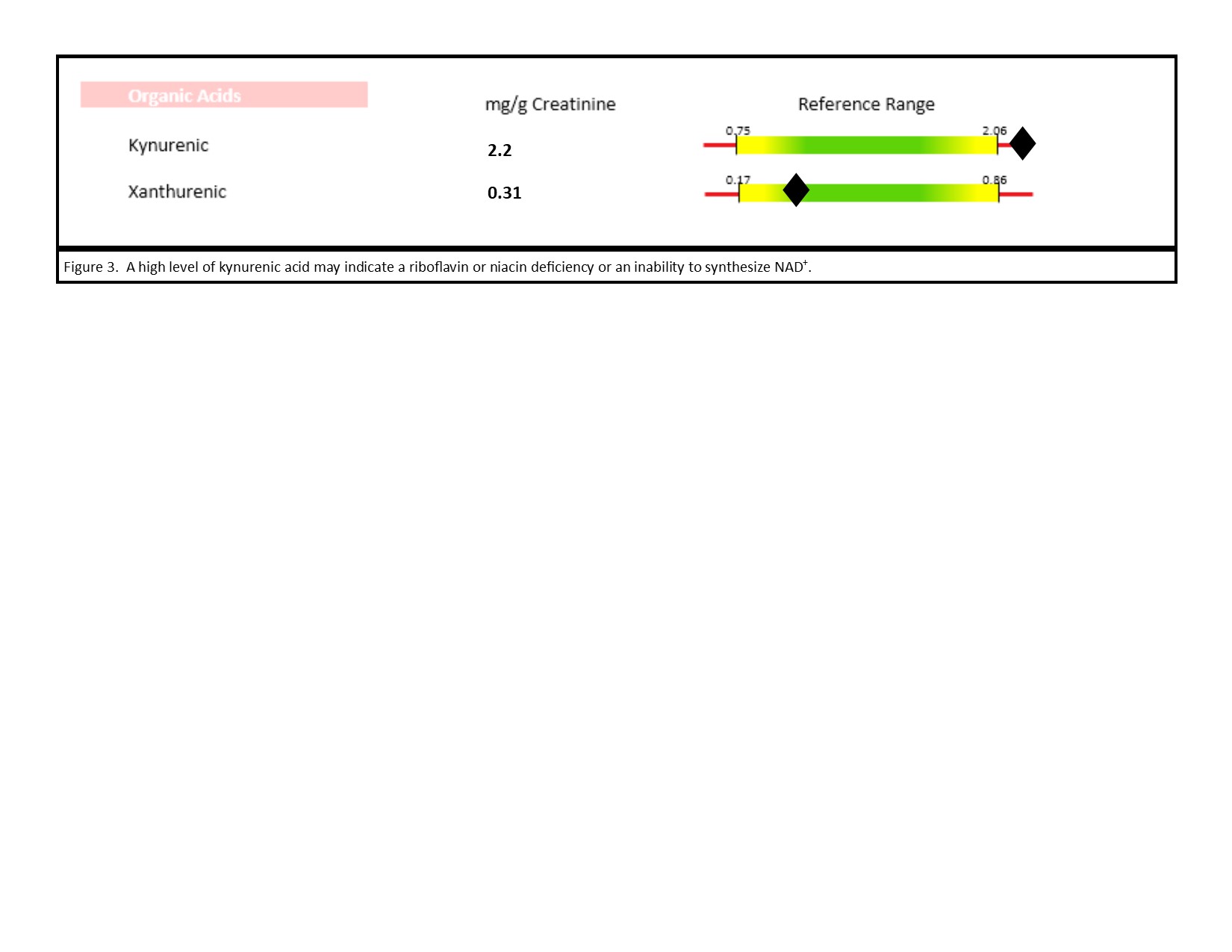
The HPA axis may also be disrupted by toxic exposures. Blood levels of cortisol are monitored by the hypothalamus in the brain.35 When blood cortisol levels decline, the hypothalamus releases CRH and arginine vasopressin to activate the HPA axis. The pituitary, when stimulated by CRH, releases ACTH, which stimulates the adrenal gland to produce and release cortisol. Environmentally induced signaling failures in the HPA axis may contribute to adrenal insufficiency.2,30 Cadmium exposure has been shown to alter pituitary function and levels of prolactin, ACTH, thyroid-stimulating hormone (TSH), and growth hormone in animal studies.3 Lead has been shown to reduce ACTH-induced glucocorticoid production in other studies.3 Toxic metal exposures can also disrupt the diurnal chronicity of pituitary signaling for both ACTH and TSH.3
Receptors
Toxic exposures can also alter the function or conformation of receptors, which may further alter HPA axis signaling.38 Downregulation of receptors can inhibit the effects of cortisol, resulting in a functional adrenal insufficiency – although cortisol may be produced, there are not enough cellular receptors to receive the signal correctly, resulting in an apparent hypofunction. As a result, such individuals may produce cortisol but present clinically as if they have an adrenal insufficiency (“adrenal fatigue”). These individuals are likely to respond well to detoxification protocols and stress management, as current evidence indicates that mind-body interventions are able to alter the epigenetic methylation of genes for receptors, enzymes, and inflammatory cytokines.39-41 Compounds containing antimony, barium, cadmium, lead, and tin, as well as compounds containing cobalt, copper, lithium, manganese, and zinc, are all antagonists of glucocorticoids and mineralocorticoids in vitro.42 Chemicals such as pyrethroids, organochlorines, ethiofencarb insecticide, atrazine herbicide, and tolylfluanid fungicide have antagonistic effects on glucocorticoid receptors.43 Other cell studies indicate that, even at low background levels, many chemicals have cumulative anti-mineralocorticoid effects.44 Other toxicants that can affect receptors include bacterial lipopolysaccharide (endotoxin), fungal aflatoxin, bacterial Shiga toxin, mold mycotoxins, and cigarette smoke.38
Treatment & Prevention
If environmental exposures can contribute to adrenal insufficiency, then toxicant avoidance, detoxification, and nutritional support of antioxidant and detoxification pathways may improve adrenal function, axis signaling, and hormone metabolism; this in turn may permit lower doses of replacement hormones or perhaps prevent disease progression.7,23,45,46
The first step of any detoxification protocol is the elimination of the exposure.23 Screening for recent or ongoing toxic exposures may be done using urine or blood (or hair for toxic metals); the time-frame for exposure varies by sample type, from weeks to months.7 Elimination of stored toxins is the next step. Some toxic metals may require chelation. However, toxic metals (eg, arsenic, cadmium, lead, mercury) and many lipophilic chemicals (eg, PCBs, polybrominated biphenyls, chlorinated pesticides, hexachlorobenzene) may be excreted in human sweat.47-49 Sweating combined with weight loss has been shown to be an efficient method of reducing a chemical body burden. The bacteria of the gut microbiome possess many classes of metabolic and detoxification enzymes and may thus perform many of the same biochemical transactions as the human liver. A diet rich in fruits, vegetables, and other fibers has been shown to improve microbial diversity and decrease enterohepatic recirculation and circulating endotoxin.28,50,51 A diverse microbiome may improve autoimmune diseases through the promotion of immune tolerance and anti-inflammatory cell-signaling.52,53 Increasing the amount of plant-based foods in the diet also increases the amount of dietary antioxidant and anti-inflammatory phytochemicals, which can aid detoxification.54
Nutritional Support
Nutritional support of biochemical detoxification processes, mitochondrial functions, and antioxidant status is the next step. Liver dysfunction has the potential to impair cortisol metabolism and alter a hormone’s half-life.55 Different toxicants may increase or decrease the activity of specific liver enzymes and affect the levels of glucocorticoids, mineralocorticoids, or sex hormones.56
- Phase I detoxification is performed by CYP450 oxidase, reductase, and dehydrogenase enzymes that convert fat-soluble compounds into water-soluble compounds for elimination from the body.57 Each CYP450 enzyme requires a heme molecule, so anemic patients must be evaluated, and the cause corrected, prior to initiating a detoxification program.58,59 Support Phase I with selenium, zinc, and vitamins A, D, E, K, and C. Plant compounds, such as sulforaphanes found in broccoli and other Brassica family vegetables, also support Phase I detoxification.54,60
- The primary Phase II pathways for hormones are glucuronidation, sulfonation, and methylation.54,61 All 3 pathways may be influenced by inheritance or overwhelmed by toxic exposures, and 2 of the pathways are supported by nutrient supplementation.
- The glucuronidation pathway may be supported by the addition of calcium-D-glucarate or D-glucaric acid62 to improve enzyme function, and early animal evidence indicates that calorie restriction may upregulate glucuronidation enzymes.63
- Phase II methylation occurs via methyltransferase enzymes, including catechol-O-methyltransferase (COMT; B6, magnesium, S-adenosylmethionine [SAMe] are cofactors) (Figure 4).64 These enzymes are part of a functional methylation cycle, and make a toxic byproduct, S-adenosylhomocysteine (SAH). High SAH levels may contraindicate the use of SAMe, as SAH is an oxidative stressor that contributes to cardiovascular and inflammatory disease.65,66 Prior to using supplemental SAMe, consider confirming the individual’s ability to metabolize SAMe via a functional blood analysis of the methylation pathway.
- Phase III detoxification occurs when a metabolized xenobiotic is transported out of the cell for excretion in urine or bile.67 Nutritional support of Phase III efflux pumps includes mitochondrial support nutrients such as B vitamins, magnesium, manganese, iron, a-lipoic acid and CoQ10.68-70
Figure 4. Methylated Estrogen Metabolites
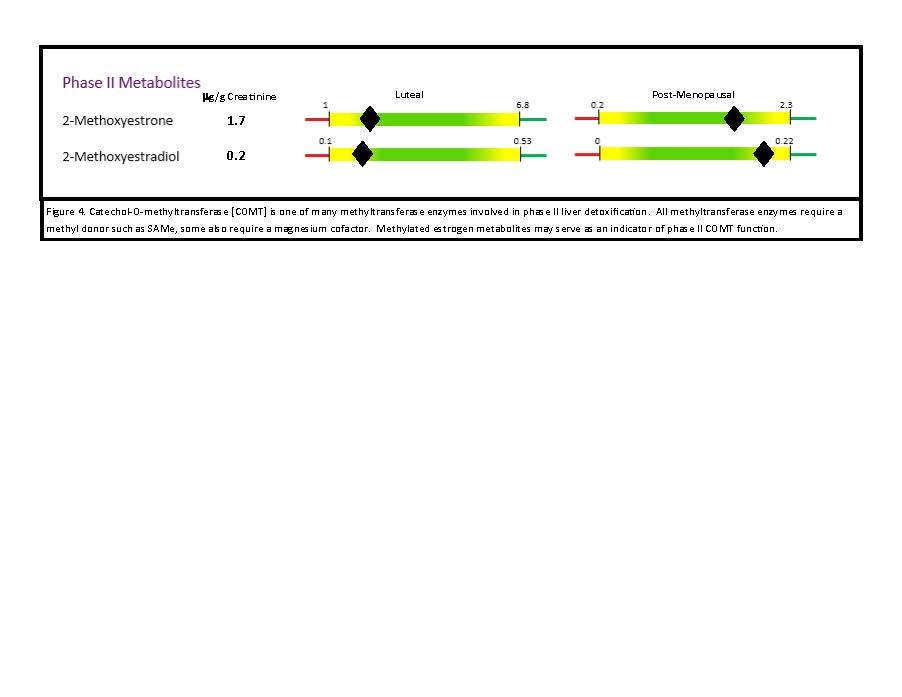
Adrenal Support
Supporting the adrenal glands is also important. Adrenal function may be supported with nutrients essential for cortisol synthesis, such as potassium, vitamins B3 and B5, and ascorbic acid.71-75 Most adaptogenic herbs (eg, Panax spp, Withania somnifera, Eleutherococcus senticosus) do not directly alter cortisol levels, but rather appear to alter cell-signaling pathways or increase dehydroepiandrosterone (DHEA).76 Other herbal support to consider includes Cordyceps sinensis (or C militaris), which has been shown in animal studies to induce the conversion of cholesterol to the hormone precursor pregnenolone and to increase corticosterone synthesis.77,78
Conclusion
Naturopathic medicine, with its emphasis on detoxification and treatment of causative factors, has much to offer individuals diagnosed with adrenal insufficiency. The increased oxidative stress and decreased enzyme activity induced by toxic exposures, combined with poor nutrition and inherited variations in enzymes of steroid hormone metabolism and detoxification, can contribute to adrenal hypofunction in susceptible individuals. Relieving the burden may prevent the progression to autoimmunity, as well as improve adrenal function in those at risk for environmentally-induced adrenal hypofunction.
References:
- Dunlop BW, Wong A. The hypothalamic-pituitary-adrenal axis in PTSD: Pathophysiology and treatment interventions. Prog Neuropsychopharmacol Biol Psychiatry. 2019;89:361-379.
- Cadegiani FA, Kater CE. Adrenal fatigue does not exist: a systematic review. BMC Endocr Disord.2016;16(1):48.
- Rana SV. Perspectives in endocrine toxicity of heavy metals–a review. Biol Trace Elem Res.2014;160(1):1-14.
- Sanderson JT. The steroid hormone biosynthesis pathway as a target for endocrine-disrupting chemicals. Toxicol Sci. 2006;94(1):3-21.
- Harvey PW, Everett DJ. The adrenal cortex and steroidogenesis as cellular and molecular targets for toxicity: critical omissions from regulatory endocrine disrupter screening strategies for human health? J Appl Toxicol.2003;23(2):81-87.
- Neier K, Marchlewicz EH, Dolinoy DC, Padmanabhan V. Assessing Human Health Risk to Endocrine Disrupting Chemicals: a Focus on Prenatal Exposures and Oxidative Stress. Endocr Disruptors (Austin). 2015;3(1). pii: e1069916. [Epub 2015 Jul 28.]
- Sears ME, Genuis SJ. Environmental determinants of chronic disease and medical approaches: recognition, avoidance, supportive therapy, and detoxification. J Environ Public Health. 2012;2012:356798.
- Genuis SJ, Tymchak MG. Approach to patients with unexplained multimorbidity with sensitivities. Can Fam Physician.2014;60(6):533-538.
- Cohen S, Schwartz JE, Epel E, et al. Socioeconomic status, race, and diurnal cortisol decline in the Coronary Artery Risk Development in Young Adults (CARDIA) Study. Psychosom Med. 2006;68(1):41-50.
- Dmitrieva NO, Almeida DM, Dmitrieva J, et al. A day-centered approach to modeling cortisol: diurnal cortisol profiles and their associations among U.S. adults. Psychoneuroendocrinology. 2013;38(10):2354-2365.
- Liao J, Brunner EJ, Kumari M. Is there an association between work stress and diurnal cortisol patterns? Findings from the Whitehall II study. PLoS One. 2013;8(12):e81020.
- Schreier HM, Hsu HH, Amarasiriwardena C, et al. Mercury and psychosocial stress exposure interact to predict maternal diurnal cortisol during pregnancy. Environ Health. 2015;14:28.
- Michels A, Michels N. Addison disease: early detection and treatment principles. Am Fam Physician. 2014;89(7):563-568.
- Betterle C, Garelli S, Presotto F, Furmaniak J. From Appearance of Adrenal Autoantibodies to Clinical Symptoms of Addison’s Disease: Natural History. Front Horm Res.2016;46:133-145.
- Gambelunghe G, Kockum I, Bini V, De Giorgi G, Celi F, et al. Retrovirus-like long-terminal repeat DQ-LTR13 and genetic susceptibility to type 1 diabetes and autoimmune Addison’s disease. Diabetes.2005;54(3):900-905.
- Martinez-Arguelles DB, Papadopoulos V. Mechanisms mediating environmental chemical-induced endocrine disruption in the adrenal gland. Front Endocrinol (Lausanne). 2015;6:29.
- New MI. Addison’s Disease. Last updated 2018. National Organization for Rare Disorders. Available at: https://rarediseases.org/rare-diseases/addisons-disease/. Accessed March 29, 2019.
- Grossman AB. Secondary Adrenal Insufficiency. Last revised July 2019. Merck Manual Web site. http://www.merckmanuals.com/professional/endocrine-and-metabolic-disorders/adrenal-disorders/secondary-adrenal-insufficiency. Accessed August 21, 2017.
- Sahakitrungruang T. Clinical and molecular review of atypical congenital adrenal hyperplasia. Ann Pediatr Endocrinol Metab. 2015;20(1):1-7.
- Andreoli V, Sprovieri F. Genetic Aspects of Susceptibility to Mercury Toxicity: An Overview. Int J Environ Res Public Health. 2017;14(1). pii: E93. doi: 10.3390/ijerph14010093.
- Heim C, Ehlert, U, Hellhammer DH. The potential role of hypocortisolism in the pathophysiology of stress-related bodily disorders. Psychoneuroendocrinology. 2000;25(1):1-35.
- Jeanneteau F, Arango-Lievano M. Linking Mitochondria to Synapses: New Insights for Stress-Related Neuropsychiatric Disorders. Neural Plast. 2016;2016:3985063.
- Genuis SJ. Elimination of persistent toxicants from the human body. Hum Exp Toxicol. 2011;30(1):3-18.
- Baxter M, Gorick S, Swords FM. Recovery of adrenal function in a patient with confirmed Addison’s disease. Endocrinol Diabetes Metab Case Rep.2013;2013:130070.
- Miller FW, Alfredsson L, Costenbader KH, et al. Epidemiology of environmental exposures and human autoimmune diseases: findings from a National Institute of Environmental Health Sciences Expert Panel Workshop. J Autoimmun. 2012;39(4):259-271.
- Pollard KM, Hultman P, Kono DH. Toxicology of autoimmune diseases. Chem Res Toxicol.2010;23(3):455-466.
- Iavicoli I, Fontana L, Bergamaschi A. The effects of metals as endocrine disruptors. J Toxicol Environ Health B Crit Rev. 2009;12(3):206-223.
- Guengerich FP. Intersection of Roles of Cytochrome P450 Enzymes with Xenobiotic and Endogenous Substrates. Relevance to Toxicity and Drug Interactions. Chemical Res Toxicol. 2017;30(1):2-12.
- Meyer JN, Leung MC, Rooney JP, et al. Mitochondria as a Target of Environmental Toxicants. Toxicol Sci. 2013;134(1):1-17.
- Harvey PW, Everett DJ, Springall CJ. Adrenal toxicology: a strategy for assessment of functional toxicity to the adrenal cortex and steroidogenesis. J Appl Toxicol. 2007;27(2):103-115.
- Ullerås E, Ohlsson Å, Oskarsson A. Secretion of cortisol and aldosterone as a vulnerable target for adrenal endocrine disruption – screening of 30 selected chemicals in the human H295R cell model. J Appl Toxicol. 2008;28(8):1045-1053.
- Michael Caudle W. This can’t be stressed enough: The contribution of select environmental toxicants to disruption of the stress circuitry and response. Physiol Behav.2016;166:65-75.
- Vaiserman A. Early-life Exposure to Endocrine Disrupting Chemicals and Later-life Health Outcomes: An Epigenetic Bridge? Aging Dis. 2014;5(6):419-429
- Pozo OJ, Marcos J, Fabregat A, et al. Adrenal hormonal imbalance in acute intermittent porphyria patients: results of a case control study. Orphanet J Rare Dis. 2014;9:54.
- Chapman K, Holmes M, Seckl J. 11β-hydroxysteroid dehydrogenases: intracellular gate-keepers of tissue glucocorticoid action. Physiol Rev.2013;93(3):1139-1206.
- Bánhegyi G, Csala M, Benedetti A. Hexose-6-phosphate dehydrogenase: linking endocrinology and metabolism in the endoplasmic reticulum. J Mol Endocrinol. 2009;42(4):283-289.
- Zhou CC, Yang X, Hua X, et al. Hepatic NAD(+) deficiency as a therapeutic target for non-alcoholic fatty liver disease in ageing. Br J Pharmacol. 2016;173(15):2352-2368.
- Marketon JI, Sternberg EM. The glucocorticoid receptor: a revisited target for toxins. Toxins. 2010;2(6):1357-1380.
- Tyrka AR, Price LH, Marsit C, et al. Childhood adversity and epigenetic modulation of the leukocyte glucocorticoid receptor: preliminary findings in healthy adults. PloS One. 2012;7(1):e30148.
- Bishop JR, Lee AM, Mills LJ, et al. Methylation of FKBP5 and SLC6A4 in Relation to Treatment Response to Mindfulness Based Stress Reduction for Posttraumatic Stress Disorder. Front Psychiatry. 2018;9:418.
- Buric I, Farias M, Jong J, et al. What Is the Molecular Signature of Mind-Body Interventions? A Systematic Review of Gene Expression Changes Induced by Meditation and Related Practices. Front Immunol. 2017;8:670.
- Zhang J, Yang Y, Liu W, Liu J. Potential endocrine-disrupting effects of metals via interference with glucocorticoid and mineralocorticoid receptors. Environ Pollut.2018;242(Pt A):12-18.
- Zhang J, Zhang J, Liu R, et al. Endocrine-Disrupting Effects of Pesticides through Interference with Human Glucocorticoid Receptor. Environ Sci Technol. 2016;50(1):435-443.
- Zhang J, Huang X, Liu H, et al. Novel Pathways of Endocrine Disruption Through Pesticides Interference with Human Mineralocorticoid Receptors. Toxicol Sci. 2018;162(1):53-63.
- Sargis RM, Heindel JJ, Padmanabhan V. Interventions to Address Environmental Metabolism-Disrupting Chemicals: Changing the Narrative to Empower Action to Restore Metabolic Health. Front Endocrinol (Lausanne).2019;10:33.
- Quinkler M, Beuschlein F, Hahner S, et al. Adrenal cortical insufficiency-a life threatening illness with multiple etiologies. Dtsch Arztebl Int.2013;110(51-52):882-888.
- Hussain J, Cohen M. Clinical Effects of Regular Dry Sauna Bathing: A Systematic Review. Evidence Based Complement Alternat Med. 2018;2018:1857413.
- Genuis SJ, Birkholz D, Rodushkin I, Beesoon S. Blood, urine, and sweat (BUS) study: monitoring and elimination of bioaccumulated toxic elements. Arch Environ Contam Toxicol. 2011;61(2):344-357.
- Sears M, Kerr K, Bray R. Arsenic, cadmium, lead, and mercury in sweat: a systematic review. J Environ Public Health.2012;2012:184745.
- Koppel N, Maini Rekdal V, Balskus EP. Chemical transformation of xenobiotics by the human gut microbiota. Science. 2017;356(6344). pii: eaag2770. doi: 10.1126/science.aag2770.
- Saltzman ET, Palacios T, Thomsen M, Vitetta L. Intestinal Microbiome Shifts, Dysbiosis, Inflammation, and Non-alcoholic Fatty Liver Disease. Front Microbiol.20189:61.
- Committee on Advancing Understanding of the Implications of Environmental-Chemical Interactions with the Human Microbiome. Environmental Chemicals, the Human Microbiome, and Health Risk: A Research Strategy. National Academies Press (US); 2017 Dec 29. Washington (DC). Available at: https://www.ncbi.nlm.nih.gov/books/NBK481561/#sec_000021. Accessed March 6, 2019.
- Opazo MC, Ortega-Rocha EM, Coronado-Arrázola I, et al. Intestinal Microbiota Influences Non-intestinal Related Autoimmune Diseases. Front Microbiol. 2018;9:432.
- Hodges RE, Minich DM. Modulation of Metabolic Detoxification Pathways Using Foods and Food-Derived Components: A Scientific Review with Clinical Application. J Nutr Metab. 2015;2015:760689.
- Nebert DW, Wikvall K, Miller WL. Human cytochromes P450 in health and disease. Philos Trans R Soc Lond B Biol Sci. 2013;368(1612):20120431.
- Niwa T, Murayama N, Imagawa Y, Yamazaki H. Regioselective hydroxylation of steroid hormones by human cytochromes P450. Drug Metab Rev. 201547(2):89-110.
- Grant DM. Detoxification pathways in the liver. J Inherit Metab Dis. 1991;14(4):421-430.
- MA, Sinclair PR, De Matteis F. Cytochrome P450 regulation: the interplay between its heme and apoprotein moieties in synthesis, assembly, repair and disposal. Drug Metab Rev. 2011;43(1):1-26.
- Besur S, Hou W, Schmeltzer P, Bonkovsky HL. Clinically important features of porphyrin and heme metabolism and the porphyrias. Metabolites. 2014;4(4):977-1006.
- Pandey KB, Rizvi SI. Plant polyphenols as dietary antioxidants in human health and disease. Oxid Med Cell Longev. 2009;2(5):270-278.
- Sarkar U, Rivera-Burgos D, Large EM, et al. Metabolite Profiling and Pharmacokinetic Evaluation of Hydrocortisone in a Perfused Three-Dimensional Human Liver Bioreactor. Drug Metab Dispos. 2015;43(7):1091-1099.
- Examine.com. Summary of Calcium-D-Glucarate. Available at: https://examine.com/supplements/calcium-d-glucarate/#ref5. Accessed March 6, 2019.
- Wen H, Yang HJ, An YJ, et al. Enhanced phase II detoxification contributes to beneficial effects of dietary restriction as revealed by multi-platform metabolomics studies. Mol Cell Proteomics.2013;12(3):575-586.
- Hevir N, Šinkovec J, Rižner TL. Disturbed expression of phase I and phase II estrogen-metabolizing enzymes in endometrial cancer: lower levels of CYP1B1 and increased expression of S-COMT. Mol Cell Endocrinol.2011;331(1):158-167.
- Škovierová H, Vidomanová E, Mahmood S, et al. The Molecular and Cellular Effect of Homocysteine Metabolism Imbalance on Human Health. Int J Mol Sci. 2016;17(10). pii: E1733.
- Xiao Y, Su X, Huang W, et al. Role of S-adenosylhomocysteine in cardiovascular disease and its potential epigenetic mechanism. Int J Biochem Cell Biol. 2015;67:158-166.
- Zimniak P, Awasthi S, Awasthi YC. Phase III detoxification system. Trends Biochem Sci. 1993;18(5):164-166.
- Vasiliou V, Vasiliou K, Nebert DW. Human ATP-binding cassette (ABC) transporter family. Hum Genomics. 2009;3(3):281-290.
- Liesa M, Qiu W, Shirihai OS. Mitochondrial ABC transporters function: the role of ABCB10 (ABC-me) as a novel player in cellular handling of reactive oxygen species. Biochim Biophys Acta. 2012;1823(10):1945-1957.
- Nicolson GL. Mitochondrial Dysfunction and Chronic Disease: Treatment With Natural Supplements. Integr Med (Encinitas). 2014;13(4):35-43.
- Patak P, Willenberg HS, Bornstein SR. Vitamin C is an important cofactor for both adrenal cortex and adrenal medulla. Endocr Res. 2004;30(4):871-875.
- Ueda Y, Honda M, Tsuchiya M, et al. Response of plasma ACTH and adrenocortical hormones to potassium loading in essential hypertension. Jpn Circ J. 1982;46(4):317-322.
- van Heeswijk RP, Cooper CL, Foster BC, et al. Effect of high-dose vitamin C on hepatic cytochrome P450 3A4 activity. Pharmacotherapy. 2005;25(12):1725-1728.
- Chan S, Debono M. Replication of cortisol circadian rhythm: new advances in hydrocortisone replacement therapy. Ther Adv Endocrinol Metab. 2010;1(3):129-138.
- Camfield DA, Wetherell MA, Scholey AB, et al. The effects of multivitamin supplementation on diurnal cortisol secretion and perceived stress. Nutrients. 2013;5(11):4429-4450.
- Panossian A, Wikman G. Evidence-based efficacy of adaptogens in fatigue, and molecular mechanisms related to their stress-protective activity. Curr Clin Pharmacol. 2009;4(3):198-219.
- Leu SF, Poon SL, Pao HY, Huang BM. The in vivo and in vitro stimulatory effects of cordycepin on mouse leydig cell steroidogenesis. Biosci Biotechnol Biochem.2011;75(4):723-731.
- Leu S, Chien C, Tseng C, et al. The in vivo effect of Cordyceps sinensis mycelium on plasma corticosterone level in male mouse. Biol Pharm Bull.2005;28(9):1722-1725.
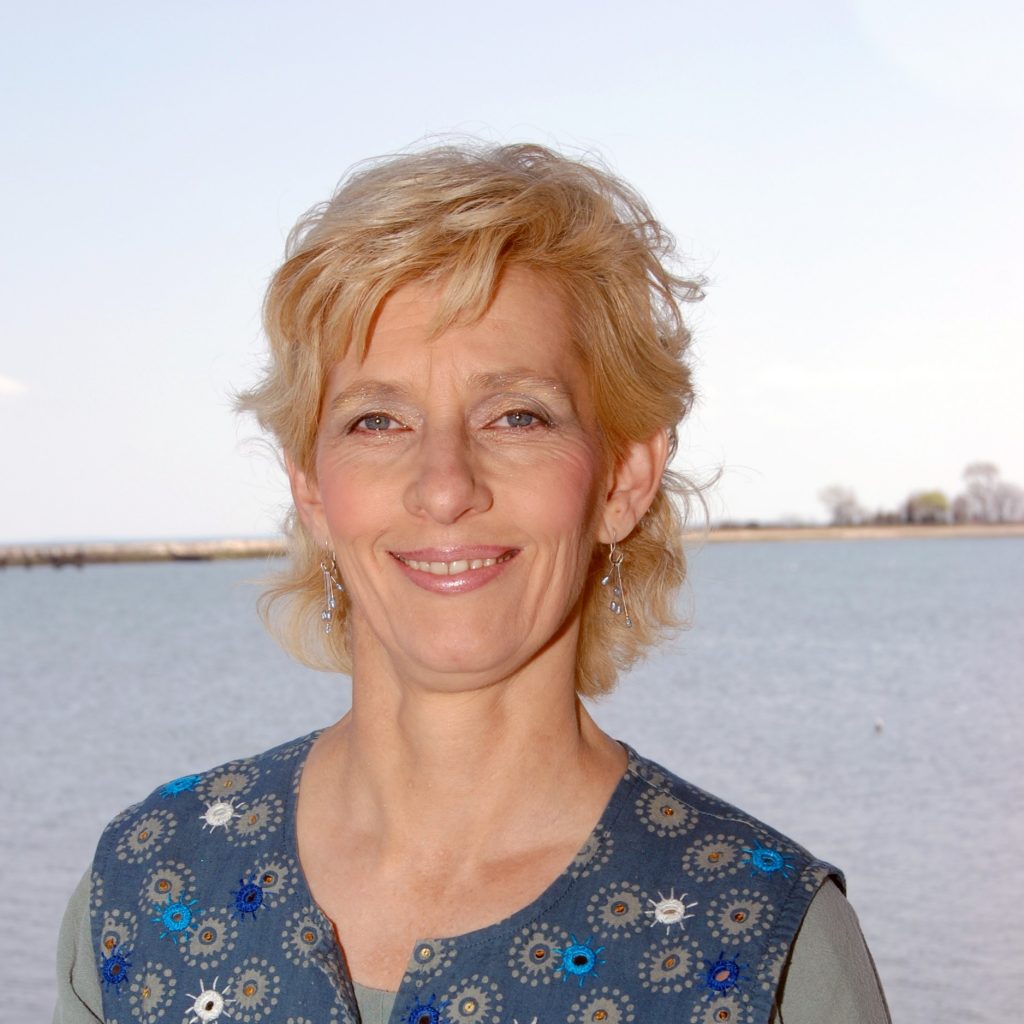
Andrea Gruszecki, ND, received her BA in Ecology and Evolutionary Biology from the University of CT, and her doctorate in Naturopathy from SCNM (2000). Trained as a radiologic technologist and Army medic, she spent the years prior to graduation working in urgent care and hospital settings, gaining valuable clinical experience. Dr Gruszecki is a member of the Consulting Department at Meridian Valley Laboratory, where she provides interpretive assistance with laboratory results, writes interpretations, and creates conference presentations. She is expanding her hormonal knowledge, and brings her expertise in urinary neurotransmitters, toxic metals, and methylation pathways to the interpretation of MVL laboratory results.