Gut Microbiota & CA Treatment: New Research and Clinical Relevance
Katherine Hampilos
Wendy Hodsdon, ND
The gut microbiota, made up of trillions of microorganisms that colonize the distal digestive tract, is required for the development and persistence of a healthy immune response.1 Intestinal immune maturation is dependent on the presence of commensal bacteria for the development of Peyer’s patches, the production of plasma cells and intraepithelial lymphocytes, and the secretion of functional immunoglobulin A (IgA).2 Gut bacteria have also been shown to influence the mature immune system by conditioning mononuclear phagocytes and shaping T-cell responses in the intestine. Next-generation sequencing has allowed scientists to characterize the composition of the gut microbiota on an individual level, and has revealed that gut bacterial communities differ significantly between healthy and diseased individuals.3 Currently, a large body of research exists demonstrating the association between an abnormal microbiota – termed dysbiosis – and the development of various types of cancer, including oral squamous cell carcinoma, pancreatic cancer, and colorectal cancer.4 However, very little is known about the role of the gut microbiome in cancer treatment and tumor eradication. This review evaluates recent in vivo research on the role of the microbiome in conventional cancer treatment and discusses the clinical implications for the future of both conventional and adjunctive cancer care.
Cytotoxic drugs target rapidly dividing cancer cells and induce apoptosis; however, these agents often have low specificity for cancer cells and also damage other rapidly proliferating healthy host tissue. Many chemotherapeutic agents infiltrate the bone marrow and decrease white blood cell counts, in particular neutrophils, putting patients at increased risk of bacterial infection.5 As a result, antibiotic prophylaxis is recommended in high-risk patients to prevent neutropenic sepsis. One systematic review of patients with hematologic malignancies revealed that prophylactic antibiotics significantly decreased all-cause mortality in patients undergoing chemotherapy compared to no intervention, with a number needed to treat of 34.5 However, in low-risk patients with solid tumors, antibiotic prophylaxis is not routinely recommended due to the lack of demonstrable benefit and the risk of antibiotic resistance.6 While additional clinical research is needed before any definitive conclusions can be drawn, recent research supports the use of caution in prescribing prophylactic antibiotics with chemotherapy, as antibiotic administration may disrupt the complex interplay between the microbiota and the immune response to cancer in humans.
New Research
Two major studies, both published in 2013, were the first to examine the influence of the microbiota on the efficacy of various conventional cancer treatments.7,8 These studies compared tumor progression in mice raised in a germ-free environment (GF) and mice treated with prophylactic broad-spectrum antibiotics (ABX) to control mice with intact intestinal microbiota. One study found that that the gut microbiota is involved in regulating the immune response to cancer at the level of gene expression in tumor cells.7 Gene analysis of untreated tumor in both groups revealed down-regulation of genes involved in inflammation, phagocytosis, antigen presentation, and adaptive immune response and up-regulation of genes involved in tissue development and cancer metabolism.
Iida et al investigated whether the presence of an intact gut microbiota modulates the overall efficacy of a combined anti-cancer immunotherapy regimen used in humans to slow tumor growth.7 This immunotherapy combination uses CpG containing oligodeoxynucleotides (CpG-ODN) and interleukin (IL)-10 receptor antibodies (IL10R) to induce both a CD8+ T-cell response and an increased production of tumor necrosis factor-alpha (TNF-α) by tumor-associated myeloid cells.9 Iida et al found that prophylactic treatment with ABX significantly impaired the ability of this immunotherapy regimen to reduce tumor burden and prevent death in mice with transplantable tumors. Further analysis revealed that the mechanism by which ABX interfere with this immunotherapy regimen is by reducing overall TNF-α production. In mice with intact microbiota, Iida et al found that specific gram-negative commensal bacteria (Alistipes) and gram-positive bacteria (Rumonicoccus) were associated with increased TNF-α production, while other genera (Lactobacillus) were associated with decreased TNF-α production. This research indicates that specific bacterial genera are involved in priming tumor-associated myeloid cells for inflammatory cytokine production in response to CpG-ODN/IL10R immunotherapy.
Viaud et al explored the influence of the microbiome on the efficacy of cyclophosphamide, a common chemotherapeutic alkylating agent.8 Low-dose cyclophosphamide is used in cancer patients to promote anti-cancer Th1 and Th17 cells.10 The findings from Viaud et al revealed that in tumor-bearing mice, ABX treatment attenuates the ability of cyclophosphamide to polarize immune cells toward a Th1/Th17 response and thus blunts its anti-tumor effects.8 Cyclophosphamide was shown to enhance the permeability of the small intestine and induce the translocation of specific species of gram-positive bacteria into secondary lymphoid organs. Once there, the bacteria were able to polarize CD4+ T helper cells into a Th1 or Th17 phenotype and to increase their production of interferon gamma (IFN-γ) and IL-17, respectively. This effect was dependent on the gut microbiota and was not seen in mice treated prophylactically with ABX or GF mice. Further analysis revealed that a specific set of gram-positive commensal bacteria, Lactobacillus johnsonii and Enterococcus hirae, were necessary to mediate the cyclophosphamide-driven accumulation of Th1 and Th17 memory T cells. Finally, an oral lavage of L johnsonii and E hirae given to ABX-treated mice restored the ability of cyclophosphamide to promote Th17 cells in the spleen.8 It is clear from these findings that specific beneficial bacteria play a crucial role in the efficacy of cyclophosphamide by modulating the systemic T-cell response to cancer.
Finally, Iida et al demonstrated that the microbiome also influences chemotherapeutics that do not directly interact with cells of the adaptive immune system.7 Platinum compounds cisplatin and oxaliplatin induce apoptosis by causing DNA crosslinking and inhibiting DNA repair and DNA synthesis in cancer cells.11 They also induce reactive oxygen species (ROS) production as a mediator of apoptosis and an important aspect of their anticancer activity. Iida et al revealed that GF and ABX-treated mice failed to respond to both cisplatin and oxaliplatin treatment.7 Further analysis demonstrated that while the levels of tumor DNA-bound platinum was the same in all groups, only GF and ABX mice exhibited reduced expression of genes Nox1 and Cybb that encode NADPH oxidase 2, an enzyme that generates ROS. This finding indicates that the gut microbiota can also increase tumor-associated myeloid cell ROS production and influence cytotoxic treatments independent of T cells or cytokines.
Taken together, the results of these studies demonstrate that gut bacteria influence chemotherapeutic drug efficacy through various mechanisms, including myeloid cell function, genetic transcription, and T-cell proliferation. These findings imply that prophylactic antibiotic treatment may reduce the efficacy of some chemotherapeutic agents in humans by suppressing the ability of the microbiota to influence the systemic immune response. Though additional studies in humans are necessary before any definitive conclusions can be drawn, these findings do have numerous implications for the current standard of care surrounding chemotherapy treatment.
Considerations for Adjunctive Cancer Care
Instead of using antibiotics to kill bacteria indiscriminately, physicians could instead focus their efforts on restoring an optimal microbial composition prior to chemotherapy administration. The studies described above indicate that the presence of specific commensal bacteria may increase the efficacy of different chemotherapeutic drug regimens. For example, Alistipes and Ruminococcus appear to increase the efficacy of CpG-ODN/IL10R immunotherapy,7 while L johnsonii and E hirae facilitate the action of cyclophosphamide.8 It is therefore plausible that the use of natural therapies to expand specific bacterial genera may increase the efficacy of chemotherapy agents in patients with cancer. Additionally, pre-treatment screening of the microbiota may allow physicians to determine which patients are good candidates for a particular chemotherapy regimen based on their individual microbial composition.
Supporting a healthy microbiome throughout chemotherapy treatment may also play a crucial role in both the extent of chemotherapy success and the severity of its side effects. Chemotherapy itself has been shown to alter the intestinal microbiota composition and allow pathogenic strains of bacteria to multiply on the damaged mucosal surface, leading to bacterial translocation across the gut wall and systemic exposure to bacterial products.12 According to Viaud et al, this bacterial translocation is required for cyclophosphamide to effectively promote an anti-tumor Th1/Th17 response in the secondary lymphoid organs.8 However, the bacterial genera identified by Viaud et al as priming immune cells included L johnsonii and E hirae, while the species that generally proliferate in response to chemotherapy treatment in humans include E coli and Salmonella spp.13 Preventing chemotherapy-induced dysbiosis by supporting commensal bacteria throughout treatment may therefore prevent pathologic bacteria from multiplying and allow beneficial bacteria to translocate into the lymphoid organs and elicit an immune response.
There is clearly an emerging medical need for tools to diagnose and treat microbial dysbiosis in cancer patients, both to improve the efficacy and to reduce the adverse effects of chemotherapy (Figure 1). Naturopathic physicians use a variety of modalities to manage dysbiosis, including therapeutic diet, pre- and probiotics, botanical medicine, and fecal microbiota transfer, all of which may play a role in the future of adjunctive cancer care. Currently, little to no research exists on the safety or efficacy of these treatments in cancer patients. However, probiotics have been studied extensively for their ability to reduce common side effects of chemotherapy, and serve as an example of the possible benefits of restoring the gut microbiome integrity and diversity prior to chemotherapy administration.
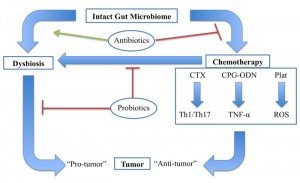
Gut microbiota modulates an antitumor response through various chemotherapeutic agents, whereas dysbiosis promotes tumor development. Antibiotics promote dysbiosis in humans and inhibit the effects of chemotherapy in mice. Probiotics may impact this system in multiple ways, including by maintaining a healthy gut microbiome and preventing chemotherapy-induced dysbiosis. Cyclophosphamide (CTX), CpG-oligodeoxynucleicid/IL-10R immunotherapy (CpG-ODN), platinum compounds cysplatin and oxaliplatin (Plat).
Figure 1. The Gut Microbiome & Cancer
Chemotherapy agents, in targeting rapidly dividing tissue, often damage the intestinal mucosa and cause debilitating mucositis. Probiotic supplementation before chemotherapy has been shown to significantly reduce the symptoms of mucositis, such as nausea, abdominal pain, and diarrhea, as well as reduce the need for anti-diarrheal medication in patients undergoing chemotherapy.14 Additionally, mucositis symptoms can be severe enough that patients require breaks in treatment that decrease overall effectiveness. In 1 open-label trial, supplementation with Lactobacillus rhamnosus not only decreased the incidence of abdominal discomfort but also decreased the need for chemotherapy dose reduction in adults treated with 5-fluorouracil compared to placebo.15 Probiotics may therefore also improve the overall efficacy of cancer treatment regimens by minimizing the need for chemotherapy dose reduction.
There are inherent risks present in intentionally administering supplemental bacteria in a population already at risk of neutropenia and infection. One systematic review examining the safety of probiotics in patients with cancer concluded that, of 17 total studies (n=1530), only 5 case reports showed possible probiotic-related positive blood cultures.14 There is also evidence in mice that probiotic supplementation with Lactobacillus casei or Lactobacillus rhamnosus actually increases immature myeloid precursors in the bone marrow as well as the early recovery of neutrophils in the blood following cyclophosphamide administration.16 While additional clinical trials are needed in cancer patients to assess the overall cost-benefit ratio, optimized probiotics remain a promising intervention for patients undergoing chemotherapy.
The relationship between the gut microbiota and the immune response to cancer is complex, though our understanding of the effects of the gut microbiome on cancer treatment is rapidly evolving. Further studies in humans are needed:
- to assess the influence of antibiotics on chemotherapy;
- to characterize the beneficial bacteria involved in promoting chemotherapy efficacy; and
- to evaluate the effects of natural treatments on the gut microbiota in patients undergoing chemotherapy
Katherine Hampilos is a third-year student at the National College of Natural Medicine in Portland, Oregon, where she is pursuing both a Doctorate in Naturopathic Medicine and a Master of Science in Integrative Medicine Research. She graduated from Oberlin College with highest honors in biology and completed her thesis in structural botany. Her current research interests include botanical medicine, integrative oncology, and rheumatology.
Wendy Hodsdon, ND, is adjunct faculty at Helfgott Research Institute at National College of Natural Medicine, where she mentors students and teaches in the Master of Science in Integrative Medicine program. Her private practice in Portland, Oregon, focuses on energy medicine and immune system disorders. She has published scientific articles and a book chapter on food allergy testing methods. More information about Dr Hodsdon can be found on her website: www.PortlandAlternativeMedicine.com.
References:
- Kabat AM, Srinivasan N, Maloy KJ. Modulation of immune development and function by intestinal microbiota. Trends Immunol. 2014;35(11):507-517.
- Kamada N, Seo SU, Chen, GY, Nuñez G. Role of the gut microbiota in immunity and inflammatory disease. Nat Rev Immunol. 2013;13(5):321-335.
- Petersen C, Round JL. Defining dysbiosis and its influence on host immunity and disease. Cell Microbiol. 2014;16(7):1024-1033.
- Bultman SJ. Emerging roles of the microbiome in cancer. 2014;35(2):249-255.
- Gafter-Gvili A, Fraser A, Paul M, et al. Antibiotic prophylaxis for bacterial infections in afebrile neutropenic patients following chemotherapy. Cochrane Database Syst Rev. 2012;1:CD004386.
- Freifeld AG, Bow EJ, Sepkowitz KA, et al. Clinical practice guideline for the use of antimicrobial agents in neutropenic patients with cancer: 2010 update by the Infectious Diseases Society of America. Clin Infect Dis. 2011;52(4):e56-e93.
- Iida N, Dzutsev A, Stweart CA, et al. Commensal bacteria control cancer response to therapy by modulating the tumor microenvironment. 2013;342(6161):967-970.
- Viaud S, Saccheri F, Mignot G, et al. The intestinal microbiota modulates the anticancer immune effects of cyclophosphamide. 2013;342(6161):971-976.
- Haining WN, Davies J, Kanzier H, et al. CpG oligodeoxynucleotides alter lymphocyte and dendritic cell trafficking in humans. Clin Cancer Res. 2008;14(17):5626-5634.
- Viaud S, Flament C, Zoubir M, et al. Cyclophosphamide induces differentiation of Th17 cells in cancer patients. Cancer Res. 2011;71(3):661-665.
- Siddik ZH. Cisplatin: mode of cytotoxic action and molecular basis of resistance. 2003;22(47):7265-7279.
- van Vliet MJ, Harmsen HJ, de Bont ES, Tissing WJ. The role of intestinal microbiota in the development and severity of chemotherapy induced mucositis. PLoS Pathog. 2010;6(5):e1000879.
- Al-Dasooqie N, Sonis ST, Bowen JM, et al. Emerging evidence on the pathobiology of mucositis. Supp Care Cancer. 2013;21(11):3233-3241.
- Redman MG, Ward EJ, Phillips RS. The efficacy and safety of probiotics in people with cancer: a systematic review. Ann Oncol. 2014;25(10):1919-1929.
- Touchefeu Y, Montassier E, Nieman K, et al. Systematic review: the role of the gut microbiota in chemotherapy- or radiation-induced gastrointestinal mucositis – current evidence and potential clinical applications. Aliment Pharmacol Ther. 2014;40(5):409-421.
- Salva S, Marranzino G, Villena J, et al. Probiotic lactobacillus strains protect against myelosupppression and immunosuppression in cyclophosphamide-treated mice. Int Immunopharm. 2014;22(1):209-221.