Endocrine Impacts of Mycotoxins
Tolle Causam
Lauren Tessier, ND
There has been a groundswell of information recently about mold, mycotoxins, and their impacts on health. Many in the allopathic community are familiar with fungal allergies and fungal infections but are much less familiar with, or even convinced of, the more controversial illness of mycotoxicosis and Chronic Inflammatory Response Syndrome (CIRS).
Mycotoxicosis can result from inhalation of environmental mycotoxins or from their consumption from food sources. Although mycotoxicosis may be largely ignored by the current medical paradigm, it certainly is not ignored by the agricultural industry, which loses an average of $932 million1 per year on mold- and mycotoxin-induced damage to our foods. As a result, a plethora of research has been published on such topics in the agricultural, zoological, and veterinary fields. Unfortunately, the research conducted to date in the human medical field is far behind, and many will use this point to support the self-interested claim that “mycotoxins are no danger to human health.” The response to this claim is 2-fold: Firstly, conducting a human study with known and intentional mycotoxin exposure would be understandably morally reprehensible in the minds of any institutional review board (IRB). Therefore, finding a double-blind randomized controlled trial (RCT) examining mycotoxin impact on human health is hard to come by. Most mycotoxin trials involving humans either examine in-vitro human cell lines or are in-vivo longitudinal studies examining the impacts of chance exposure. Second, the animals that are being examined in many of these studies are the same animals that are used in initial phases of drug trials, as their biochemistry is not unlike our own. Therefore, to ignore this data because of the population studied is to bring into question methodology of all animal-model initial drug trials. Argumentum ad consequentiam; ie, you cannot have your cake and eat it too.
A simple literature dive will demonstrate the deleterious impacts of mycotoxins on health, including immune system dysfunction and suppression, and oxidative damage leading to lipid peroxidation. Cells exposed to mycotoxins are forced to operate with altered membrane potentials, calcium homeostasis disruption, and inhibition of numerous enzymes integral to cell and organelle functionality, mitochondria included.
Mycotoxin Endocrine Effects
Compounding the harmful effects of oxidative damage is the endocrine disruption caused by mycotoxins, which results in altered metabolism2 and synthesis3 of endogenous hormones. For instance, some mycotoxins interfere with the 17-beta-hydroxysteroid dehydrogenase (HSD) class of enzymes, which are prominently involved in both androgen and estrogen metabolism.4 As many mycotoxins are also hepatotoxic, endocrine disruption may arise from aberrant hydroxylation and conjugation of other hormones. Mycotoxins can also interfere with hormone receptors, including nuclear receptors,3 by directly stimulating or blocking them.5 This can cause downstream issues with secretion, transport, and unexpected or aberrant hormonal cross-talk. The endocrine-disrupting effects of mycotoxins are not necessarily linearly dose-dependent.6 Their effects are often further altered by concentrations of the other endocrine disruptors including other mycotoxins,7 endogenous or exogenous hormones,3,6 nutritional status,8 and, potentially, stress level. Severity of endocrine disruption may differ from cell line to cell line due to variable amounts of different receptor types9 unique to each cell line.
Mycotoxins vary in their level of endocrine disruption, and each mycotoxin is present in the environment at different concentrations. For example, deoxynivalenol (DON), although a weak endocrine disruptor, is 10-100 times more prevalent in our food chain than the stronger endocrine disruptor known as zearalenone (ZEA).10 To invite further complication, mechanism of exposure should also be considered. Murine research shows that inhalation of mycotoxins may be nearly 20 times more toxic than dermal administration and 10 times more toxic than systemic administration,11 and, depending on the animal model, 2-10 times more toxic than intraperitoneal administration.12
Testosterone Disruption
As anti-aging medicine is currently in the limelight, one would be remiss to not acknowledge the disruption of sex hormones by mycotoxins. Testosterone is created in large part by the Leydig cells of the testes,13 with ancillary amounts deriving from the adrenal glands. Therefore, it is not unexpected that mycotoxins capable of inducing apoptosis of the Leydig cells, such as citrinin (CIT),14 would also be correlated with lowered testosterone levels, as shown in vitro and in animal models.6,15 In mice, Leydig cell exposure to enniatin B (ENB) results in decreased testosterone levels,16 while ENB and ZEA reduce testosterone in adrenocortical cell lines.3,16 Incubation of gonadal cells with ZEA, ochratoxin A (OTA), and CIT at differing concentrations leads to reduced testosterone secretion, with only the fusariotoxin toxin T-2 demonstrating a dose-dependent decrease.6 Meanwhile, cytochalasin B (CTB), a mycotoxin produced by select Chaetomium and Aspergillus species used in research for its anti-mitotic effect, has been shown in rats to interfere with luteinizing hormone (LH)-stimulated production of testosterone from pregnenolone at the level of the smooth endoplasmic reticulum.17 Metabolites of DON and ZEA,18 as well as beauvericin (BEA)18 also demonstrate antagonistic effects at androgen receptors in animals.
Estrogen & Progesterone Disruption
Mycotoxins are often globally categorized as xenoestrogens, but their endocrine effects go beyond estrogen alone. With that being said, mycotoxins are formidable in the realm of estrogen mimicry and agonism. The most well-known xenoestrogenic mycotoxin is ZEA; however, it should be noted that its metabolites, β-zearalenol (β-ZOL) and zearalenol (α-ZOL), are also estrogenic in nature.9 ZEA, although not a steroid, closely resembles 17ß-estradiol19 and can therefore directly bind to estrogen receptors20 and act as an agonist.21 In adrenocortical cells, ZEA, β-ZOL, and α-ZOL can increase estradiol production at low levels and decrease estradiol production at higher levels.3 Moreover, β-ZOL is able to increase estradiol levels to a greater extent than its parent mycotoxin ZEA or its fellow metabolite α-ZOL.3 A metabolite of DON, 3-acetyl-deoxynivalenol, demonstrates estrogenic activity, but to a lesser extent than β-ZOL.9 While α-ZOL demonstrates estrogenic activity approaching that of 17β-estradiol3 other studies show ZEA having a 2-3-times higher binding affinity than 17β-estradiol at the bovine endometrial estrogen receptor.22 Competition at the receptors can interfere with levels of follicle-stimulating hormone (FSH) and LH by engaging in negative feedback to the pituitary gland.23 At concentrations found in the environment, T-2 has been shown to lower FSH-stimulated estradiol production in granulosa cells.24 Alternariol, an emerging mycotoxin produced by Alternaria alternata – a common environmental mold – has been shown to increase estradiol production25 and also act as an estrogen receptor agonist.9 Meanwhile, similar to its effects on testosterone, the mycotoxin ENB has been shown to lower estradiol and progesterone in adrenocortical cell lines.16 Progesterone production may be increased by alternariol25 and decreased by ZEA in adrenal glands.3 Finally, environmental levels of T-2 appear to interfere with LH transmembrane receptor expression in human cumulus granulosa cells, while also lowering FSH-stimulated progesterone production.24
Effects on Cortisol & Thyroid
Sex hormones are not the only victims of mycotoxin-induced endocrine disruption. ZEA and ENB can both lower or raise cortisol and other adrenal hormones, depending on their concentration.3,16 CTB also interferes with cortisol release from human adrenocortical cells by reducing the action of ACTH.26 Elevations in serum cortisol result from CIT exposure in mice27; similarly, T-2 elicits plasma cortisol increases in pigs.28 In other animal studies, a mixture of T-2 and other fusarium toxins, F-2 and DAS, has been shown to cause a 200-300% rise in plasma cortisol,29 and diets containing mixed mycotoxins – including DON, ZEA, and 15-acetyl-DON (a DON metabolite) – cause elevations of serum cortisol after just 4 weeks of dietary exposure.30 As well, hyperfunctionality of the adrenal and thyroid glands was noted in newborn piglets exposed to T-2 toxin in utero.31
The thyroid is also affected by numerous mycotoxins, including aflatoxin B1 (AFB), which is well known for its hepatotoxicity and role in hepatocellular carcinoma.32 AFB also induces apoptosis in rat thyroid follicular cells,33 which are responsible for the production and secretion of thyroxine and triiodothyronine. Alternatively, in pigs, mixed fusarium mycotoxins cause a reduction in thyroid size in conjunction with an increase in total serum thyroxine levels,30 while CIT exposure in mice results in the latter in conjunction with an elevation of serum levels of triiodothyronine.27 In rats, patulin, a mycotoxin found in processed apple products, causes colloid degeneration and lymphocytic infiltration of the thyroid in addition to a decrease in thyroxine after 60 days of dietary administration.34 The dosage investigated in this study was based on estimated equivalents of dietary human exposure. BEA, OTA, and DON demonstrate antagonistic effects at the thyroid receptor,18 while ZEA alters the transcription of the alpha and beta thyroid receptors in primary cerebellar neurons.35 T-2 may exacerbate a minor iodine deficiency by interfering with the synthesis and iodination of thyroglobulin,8 potentially leading to an exacerbated hypothyroidism.
Conclusion
The endocrine-disruptive nature of mycotoxins, as evidenced by the literature is – like anything in life – not black or white. The goal of reviewing this information is not to upset or confound the reader, but rather to remind practitioners to seek the root cause when navigating hormonal imbalances. Simply supplementing hormones in a system suffering from endocrine disruption may elicit undesired effects or, ultimately, treatment failure. Proper hormonal assessment in conjunction with screening for mold and mycotoxin exposure, or any other endocrine disruptor, may help advance a relatively unresponsive case.
References:
- Council for Agricultural Science and Technology. Mycotoxins: Risks in Plant, Animal, and Human Systems. Ames, IA: Council for Agricultural Science and Technology; 2003.
- Pfeiffer E, Diwald TT, Metzler M. Patulin reduces glutathione level and enzyme activities in rat liver slices. Mol Nutr Food Res. 2005;49(4):329-336.
- Frizzell C, Ndossi D, Verhaegen S, et al. Endocrine disrupting effects of zearalenone, alpha- and beta-zearalenol at the level of nuclear receptor binding and steroidogenesis. Toxicol Lett. 2011;206(2):210-217.
- Krazeisen A, Breitling R, Möller G, Adamski J. Phytoestrogens inhibit human 17beta-hydroxysteroid dehydrogenase type 5. Mol Cell Endocrinol. 2001;171(1-2):151-162.
- Rogowska A, Pomastowski P, Sagandykova G, Buszewski B. Zearalenone and its metabolites: Effect on human health, metabolism and neutralisation methods. Toxicon. 2019;162:46-56.
- Fenske M, Fink-Gremmels J. Effects of fungal metabolites on testosterone secretion in vitro. Arch Toxicol. 1990;64(1):72-75.
- Eze UA, Huntriss J, Routledge MN, et al. The effect of individual and mixtures of mycotoxins and persistent organochloride pesticides on oestrogen receptor transcriptional activation using in vitro reporter gene assays. Food Chem Toxicol. 2019;130:68-78.
- Van Middlesworth L. T-2 mycotoxin intensifies iodine deficiency in mice fed low iodine diet. Endocrinology. 1986;118(2):583-586.
- Demaegdt H, Daminet B, Evrard A, et al. Endocrine activity of mycotoxins and mycotoxin mixtures. Food Chem Toxicol. 2016;96:107-116.
- Marin S, Ramos AJ, Cano-Sancho G, Sanchis V. Mycotoxins: occurrence, toxicology, and exposure assessment. Food Chem Toxicol. 2013;60:218-237.
- Creasia DA, Thurman JD, Jones LJ 3rd, et al. Acute inhalation toxicity of T-2 mycotoxin in mice. Fundam Appl Toxicol. 1987;8(2):230-235.
- Creasia DA, Thurman JD, Wannemacher RW Jr, Bunner DL. Acute inhalation toxicity of T-2 mycotoxin in the rat and guinea pig. Fundam Appl Toxicol. 1990;14(1):54-59.
- Adedara IA, Nanjappa MK, Farombi EO, Akingbemi BT. Aflatoxin B1 disrupts the androgen biosynthetic pathway in rat Leydig cells. Food Chem Toxicol. 2014;65:252-259.
- Liu S, Wang D, Zhang J, et al. Citrinin reduces testosterone secretion by inducing apoptosis in rat Leydig cells. Toxicol In Vitro. 2012;26(6):856-861.
- Qingqing H, Linbo Y, Yunqian G, Shuqiang L. Toxic effects of citrinin on the male reproductive system in mice. Exp Toxicol Pathol. 2012;64(5):465-469.
- Kalayou S, Ndossi D, Frizzell C, et al. An investigation of the endocrine disrupting potential of enniatin B using in vitro bioassays. Toxicol Lett. 2015;233(2):84-94.
- Murono EP, Lin T, Osterman J, Nankin HR. The effects of cytochalasin B on testosterone synthesis by interstitial cells of rat testis. Biochim Biophys Acta. 1980;633(2):228-236.
- García-Herranz V, Valdehita A, Navas JM, Fernández-Cruz ML. Cytotoxicity against fish and mammalian cell lines and endocrine activity of the mycotoxins beauvericin, deoxynivalenol and ochratoxin-A. Food Chem Toxicol. 2019;127:288-297.
- Al-Jaal BA, Jaganjac M, Barcaru A, et al. Aflatoxin, fumonisin, ochratoxin, zearalenone and deoxynivalenol biomarkers in human biological fluids: A systematic literature review, 2001-2018. Food Chem Toxicol. 2019;129:211-228.
- Kowalska K, Habrowska-Górczyńska DE, Piastowska-Ciesielska AW. Zearalenone as an endocrine disruptor in humans. Environ Toxicol Pharmacol. 2016;48:141-149.
- Malekinejad H, Colenbrander B, Fink-Gremmels J. Hydroxysteroid dehydrogenases in bovine and porcine granulosa cells convert zearalenone into its hydroxylated metabolites alpha-zearalenol and beta-zearalenol. Vet Res Commun. 2006;30(4):445-453.
- Blankenship LT, Dickey JF, Bodine AB. In vitro mycotoxin binding to bovine uterine steroid hormone receptors. Theriogenology. 1982;17(3):325-331.
- Zheng W, Feng N, Wang Y, et al. Effects of zearalenone and its derivatives on the synthesis and secretion of mammalian sex steroid hormones: A review. Food Chem Toxicol. 2019;126:262-276.
- Pogrmic-Majkic K, Samardzija Nenadov D, Stanic B, et al. T-2 toxin downregulates LHCGR expression, steroidogenesis, and cAMP level in human cumulus granulosa cells. Environ Toxicol. 2019;34(7):844-852.
- Frizzell C, Ndossi D, Kalayou S, et al. An in vitro investigation of endocrine disrupting effects of the mycotoxin alternariol. Toxicol Appl Pharmacol. 2013;271(1):64-71.
- Feuilloley M, Contesse V, Lefebvre H, et al. Effects of selective disruption of cytoskeletal elements on steroid secretion by human adrenocortical slices. Am J Physiol. 1994;266(2 Pt 1):E202-E210.
- Gupta M, Dolui AK, Dey SN, et al. Effect of the mycotoxin citrinin on some hormones and on enzymes and substrates of the Embden-Meyerhof pathway in mice. Toxicon. 1986;24(5):519-523.
- Rafai P, Tuboly S. Effect of T-2 toxin on adrenocortical function and immune response in growing pigs. Zentralbl Veterinarmed B. 1982;29(7):558-565.
- Tenk I, Fodor E, Szathmáry C. The effect of pure Fusarium toxins (T-2, F-2, DAS) on the microflora of the gut and on plasma glucocorticoid levels in rat and swine. Zentralbl Bakteriol Mikrobiol Hyg A. 1982;252(3):384-393.
- Rotter BA, Thompson BK, Lessard M, et al. Influence of low-level exposure to Fusarium mycotoxins on selected immunological and hematological parameters in young swine. Fundam Appl Toxicol. 1994;23(1):117-124.
- Ványi A, Glávits R, Gajdács E, et al. Changes induced in newborn piglets by the trichothecene toxin T-2. Acta Vet Hung. 1991;39(1-2):29-37.
- Hamid AS, Tesfamariam IG, Zhang Y, Zhang ZG. Aflatoxin B1-induced hepatocellular carcinoma in developing countries: Geographical distribution, mechanism of action and prevention. Oncol Lett. 2013;5(4):1087-1092.
- Gesing A, Jagiela J, Lewinski A. Effects of melatonin on the process of apoptosis in rat thyroid follicular cells. Neuro Endocrinol Lett. 2006;27(1-2):81-84.
- Selmanoglu G, Koçkaya EA. Investigation of the effects of patulin on thyroid and testis, and hormone levels in growing male rats. Food Chem Toxicol. 2004;42(5):721-727.
- Kiss DS, Ioja E, Toth I, et al. Comparative Analysis of Zearalenone Effects on Thyroid Receptor Alpha (TRα) and Beta (TRβ) Expression in Rat Primary Cerebellar Cell Cultures. Int J Mol Sci. 2018;19(5).
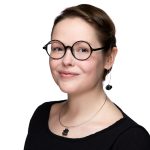
Lauren Tessier, ND, is a licensed naturopathic physician specializing in mold-related illness. She is a nationally known speaker and is Vice President of the International Society for Environmentally Acquired Illness (ISEAI) – a non-profit dedicated to educating physicians about the diagnosis and treatment of environmentally acquired illness. Dr Tessier’s practice, “Life After Mold,” in Waterbury, VT, draws clients from all around the world who suffer from chronic complex illness as a result of environmental exposure and chronic infections. Dr Tessier’s e-booklet, Mold Prevention: 101, has been widely circulated and its suggestions implemented by many worldwide.