Thyroid Health in Down Syndrome
Erica Peirson, ND
Reverse T3 in Infants with Trisomy 21
Published studies investigating thyroid function in individuals with Down syndrome date back to 1956.1 In 1958 Jerome Lejeune announced the discovery of the extra copy of chromosome 21 as the cause of Down syndrome. Even then, studies investigating thyroid function continued due to the strong correlation between the symptoms of Down syndrome and hypothyroidism. In addition, thyroid function in mothers of children with Down syndrome has even been studied as far back as 1959.2 Table 1 and Table 2 below list the symptoms that congenital hypothyroidism and newborn infants with Down syndrome have in common. Uncovering the root cause of hypothyroidism is critical to the health, development and cognition of infants and children with Down syndrome.
Given the complex nature of thyroid hormone function within the body, many Functional Medical doctors today recognize the importance of testing and evaluating all levels of thyroid hormone function. This includes thyroid stimulating hormone (TSH), free thyroxine (fT4), free triiodothyronine (fT3) and reverse triiodothyronine (rT3). Checking just TSH and fT4 is only assessing part of the process of thyroid hormone function within the body. The role of deiodinase enzymes in the thyroid hormone process cannot be ignored. These are enzymes that are responsible for the activation and deactivation of thyroid hormone. In addition, deiodinase activity occurs within the cell making it impossible to detect their activity through a simple plasma thyroid hormone level.3
Reverse T3 (3,3′, 5′-triiodothyronine) is a form of thyroid hormone that is not routinely checked in clinical practice. It is created when the deiodinase 3 enzyme removes the iodine from the inner ring of T4 (tetraiodothyronine) (Figure 1). Up-regulation of deiodinase 3 occurs in the presence of oxidative stress,7 which occurs at higher level than normal in individuals with Down syndrome even before birth. Elevated reverse T3 has been shown to interfere with active T3 function within the cell and can, therefore, create the same symptoms of hypothyroidism that a low T4 level can.4-8
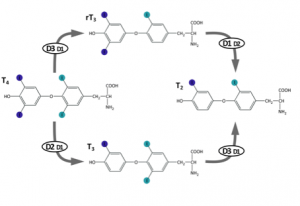
Figure 1. Thyroid hormone deiodination.
A full assessment for hypothyroidism in infants with Down syndrome includes TSH, fT4, fT3 and rT3 as well as a maintaining a high level of suspicion for hypothyroidism when delays in development and other symptoms of hypothyroidism are seen. Far too often, physicians dismiss symptoms of hypothyroidism as simply “normal for Down syndrome.”
Many researchers today are working passionately to uncover ways to improve the health and cognition of individuals with Down syndrome. Many biochemical processes are being studied including development of Alzheimer’s, circadian rhythm disruption and mitochondria function, to name a few. Disruptions in these systems being studied are almost always contributed entirely to the presence of the extra copy of chromosome 21. The role that thyroid hormone plays in these biomedical disturbances seen in individuals with Down syndrome is often ignored. Listed here are the studies that support the role that active thyroid hormone (T3) plays in the regulation of several processes that are being studied in Down syndrome.
GABA-A Maturation
Researchers from Italy are studying the use of Bumetanide, a diuretic drug that inhibits NKCC1 transporters, in a mouse model of Down syndrome.9 This sodium potassium chloride transporter is found in the brain during early development but not in adulthood. The presence of these transporters is thought to be responsible for the high intracellular chloride concentrations of very immature neurons. The shift in GABA-A receptors from excitatory to inhibitory is due to the decrease in chloride concentration within the cell as it matures.10 During early development GABA-A receptors transmit excitatory signals and in adulthood these same receptors transmit inhibitory signals as seen in Figure 2. This maturation of GABA-A receptors is an important part of normal brain development. If this maturation process doesn’t happen properly neurons are at risk for increased activation of the NKCC1 transporters which ultimately leads to their destruction via lysis. Bumetanide would prevent this destruction by inhibiting NKCC1 transporters. This maturation process has been shown to be impaired in Down syndrome and is potentially a major cause of low cognitive function in Down syndrome due to neuron destruction.11
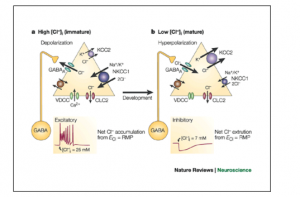
Figure 2. Excitatory actions of GABA.
It’s possible that this maturation process is impaired in Down syndrome due to untreated congenital hypothyroidism. Bumetanide would not be needed if the cause for this lack of maturation was revealed. Thyroid hormone plays a critical role in the maturation of GABA-A receptors in the brain.12,13 One important aspect of the impact that T3 hormone has on GABAergic neuron morphologic development is timing. “An early onset and continuity of T3 supplementation were necessary for the increase of burst frequency, and a late supplementation did not rescue the decreased burst frequency due to previous hormone deprivation.”13 This is a mechanism by which congenital hypothyroidism creates irreversible cognitive delay.
Amyloid Precursor Protein
The connection between Alzheimer’s disease (AD) and Down syndrome has been well-established. In fact, the rate of AD in those with Down syndrome by the age of 40 is 100%. Because no treatment for AD has been developed, identifying and treating the underlying cause is key. The most current theory behind the cause of AD is the presence of excess amyloid beta protein originating from the APP gene. Although the APP gene is on chromosome 21 and over-expression of this gene is expected, silencing of the APP gene is T3-dependent.14,15 If T3 hormone levels are not optimal, one would expect that this gene is not properly silenced. In fact, it has been shown that low levels of T3 are seen in patients with AD.16 Could the rate of AD in those with Down syndrome be reduced if T3 hormone levels were assessed and properly managed?
Sonic hedgehog
Dr. Roger Reeves and his team at Johns Hopkins University are leading the way in researching Sonic hedgehog (Shh) response deficit in Down syndrome.17-19 Shh is a protein that plays a critical role in embryonic organ development including that of the brain. The cause of Shh dysfunction in T21 has not been established. However, Desouza, et al., have shown that the Shh gene is thyroid-responsive.20 Once again, timing is important as they state, “Several studies suggest that the critical period in which thyroid hormone exerts dramatic effects on neuronal structure appears to be predominantly during early postnatal life.”
Genome-wide dysregulation
In 2014 researchers in Switzerland revealed altered gene expression throughout the entire genome of fetal Down syndrome fibroblasts.21 This new information greatly impacts the thinking that the phenotype of trisomy 21 (T21) is strictly due to genetic over-expression of chromosome 21. Dr. Ben Tycko and his team at Columbia University also showed that genome-wide impairments in methylation patterns can be seen in T lymphocytes from Down syndrome subjects.22
The variation seen in genome-wide DNA methylation patterns in T21 lymphocytes was theorized by the researchers at Columbia University to be due to the over-expression of DNMT3L gene that’s on chromosome 21. DNMT3L is the gene for one of three DNA methyltransferase that catalyzes the transfer of a methyl group to DNA. The methylation of DNA is a main epigenetic mechanism that controls DNA expression. DNMT3L controls DNA methylation through its direct action on DNMT3a and DNMT3b.23 It’s been shown that the DNMT3a gene is regulated by thyroid hormone in Xenopus tadpoles.24 Although the full extent of thyroid hormone receptor distribution is not fully understood, T3 has been shown to activate or repress genes.25 Not all T3-induced genes have been identified, yet it is recognized that thyroid hormone receptors are “widely distributed throughout the genome.”26
The question that remains unanswered is “Are the genome-wide variations seen in T21 cells due to the extra copy of chromosome 21 or is there another factor involved like T3 hormone?”
Skeletal abnormalities
While skeletal abnormalities and decreased growth in individuals with Down syndrome are often apparent when comparing adults with Down syndrome to the typical population, research supports this observation.27,28 This disturbance in normal bone growth has been linked to over-expression of Dyrk1a on chromosome 21. However, the role that thyroid hormone plays in skeletal development warrants recognition for its potential role in this impaired aspect of development seen in T21. Thyroid hormone has been shown to have a great deal of influence on fetal bone development as well as adult bone maintenance.29,30
Circadian rhythm dysregulation
Circadian rhythm disruption and sleep apnea has recently been studied for its impact on cognitive function in Down syndrome by researchers at the University of Arizona.31,32 Many parents experience sleep disruption in their children with Down syndrome that can have an impact on the overall health of the child as well as the parents. Sleep physiology is a very complicated aspect of health that requires optimal production and metabolism of neurotransmitters, optimal breathing patterns, optimal endocrine function, optimal levels of certain vitamins, among many other things. Thyroid hormone plays an important role in the body’s ability to maintain a normal circadian rhythm pattern. There are many studies linking hypothyroidism to sleep apnea and general sleep disturbance in human and animal studies.33-35
Mitochondria
The evidence of mitochondrial dysfunction in Down syndrome is strong and well-established.36-38 Symptoms of mitochondria dysfunction in children with Down syndrome include low muscle tone, slow gut motility, fatigue and delayed cognition. Mitochondria dysfunction in Down syndrome is most often attributed to oxidative stress and aberrant methylation. However, the role that thyroid hormone, both T3 and T2, plays in mitochondrial function cannot be ignored. It is thought that thyroid hormone plays a role in mitochondrial gene expression, the mitochondrial energy transduction apparatus and mitchondriogenesis.39-42 Figure 3 depicts the mechanism by which T3 and T2 modulate the respiratory chain within mitochondria.
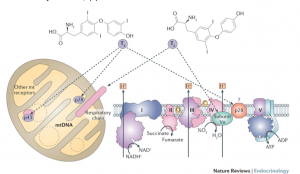
Figure 3. Davis PJ, Davis FB. Nongenomic actions of thyroid hormone. Thyroid. 1996;6(5):497–504.
Conclusion
Although there is a potential for reverse T3 playing a role in the symptoms of Down syndrome, few studies have been conducted on reverse T3 in typical newborns and infants and no studies exist comparing levels of reverse T3 in typical infants to infants with Down syndrome. In 1975 Chopra, et al concluded from their study that “high serum rT3 concentration in the newborn becomes comparable to that in the normal adult by 9-11 days of neonatal life.”43 Many years later, in 2004, Williams, et al compared cord blood levels with postnatal serum levels of thyroid hormone.44 They measured TSH, free T4, total T3, TBG and reverse T3 in order to uncover trends in postnatal serum thyroid hormone levels in preterm and term infants. They found that “The rT3 values at all postnatal ages and in all groups are substantially below the cord values of the equivalent gestational ages.” These 2 studies confirm that some knowledge exists about the potential function of reverse T3 in fetal development, but more studies are needed. Dr. Rosalind Brown has written, “This is consistent with the key role of D3 in protecting fetal tissues against high maternal T4 concentrations present either in the placenta or in amniotic fluid.”45 She acknowledges the important presence of D3, the enzyme that makes reverse T3, in fetal development.
It’s been my clinical experience after testing reverse T3 in hundreds of infants with Down syndrome is that it is elevated in a majority of infants under the age of 1-year-old. In addition, treating this elevation in reverse T3 with small doses of T3-only medication (liothyronine) in these infants alleviates their symptoms of hypothyroidism and greatly improves their development.
The current dominant medical paradigm does not recognize serum reverse T3 levels as having any function in the clinical setting. This thinking has the potential to miss a lot of patients who are experiencing hypothyroidism that is evident in many symptoms but ignored due to a patient simply having a normal TSH. Developing a better understanding of thyroid hormone dysfunction in newborns and infants with Down syndrome that could include an elevated reverse T3 can lead to better overall health, development and cognition for these children that can extend into adulthood.
Given the complexity of thyroid hormone production, activation and transport and the apparent role it may play in the cognition and health of individuals with Down syndrome more research into the mechanism behind thyroid hormone dysfunction in Down syndrome is greatly needed. As well, researchers need to be aware that when studying individuals with Down syndrome they may actually be studying the effects of hypothyroidism and not the impact of an extra copy of chromosome 21.
References:
- Cottino F, Ferraris GM, Coda G. Evaluation of certain thyroid and adrenal function values in mongolism. Folia Endocrinol Mens Incretologia Incretoterapia.1956;9(2):219-234.
- EK JI. Thyroid function in mothers of mongoloid infants. Acta Paediatr.1959;48(1):33-42.
- Bianco A. Cracking the Code for Thyroid Hormone Signaling. Trans Am Clin Climatol Assoc. 2013;124:26–35.
- Okamoto R,Leibfritz D. Adverse effects of reverse triiodothyronine on cellular metabolism as assessed by 1H and 31P NMR spectroscopy. Res Exp Med (Berl). 1997;197(4):211-217.
- Tien ES,Matsui K, Moore R, Negishi M. The nuclear receptor constitutively active/androstane receptor regulates type 1 deiodinase and thyroid hormone activity in the regenerating mouse liver. J Pharmacol Exp Ther. 2007;320(1):307-313.
- Benvenga S. A thyroid hormone binding motif is evolutionarily conserved in apolipoproteins. Thyroid. 1997;7(4):605-611.
- Santini F, Chopra IJ, Hurd RE, Solomon DH, Teco GN. A study of the characteristics of the rat placental iodothyronine 5-monodeiodinase: evidence that it is distinct from the rat hepatic iodothyronine 5′-monodeiodinase.Endocrinol. 1992;130(4):2325-2332.
- Sechman A,Niezgoda J, Sobociński R. The relationship between basal metabolic rate (BMR) and concentrations of plasma thyroid hormones in fasting cockerels. Folia Biol (Krakow). 1989;37(1-2):83-90.
- Deidda G, Parrini M, Naskar S, et al. Reversing excitatory GABAAR signaling restores synaptic plasticity and memory in a mouse model of Down syndrome.Nat Med. 2015;21:318–326.
- Galanopoulou AS. GABAAReceptors in Normal Development and Seizures: Friends or Foes? Curr Neuropharmacol. 2008;6(1):1-20.
- Smith-Hicks CL. GABAergic dysfunction in pediatric neuro-developmental disorders.Frontiers Cellular Neuroscience. 2013;7:269.
- Westerholz S, de Lima AD, Voigt T. Thyroid hormone-dependent development of early cortical networks: temporal specificity and the contribution of trkB and mTOR pathways.Front Cell Neurosci. 2013;7:121.
- Puia G, Losi G.Thyroid hormones modulate GABAA receptor-mediated currents in hippocampal neurons. Neuropharmacol. 2011;60:1254-1261.
- Belakavadi M, Dell J, Grover GJ, Fondell JD. Thyroid hormone suppression ofβ-amyloid precursor protein gene expression in the brain involves multiple epigenetic regulatory events. Mol Cell Endocrinol. 2011;339(1-2):72-80.
- Belandia B, Latasa MJ, Villa A, Pascual A. Thyroid hormone negatively regulates the transcriptional activity of the beta-amyloid precursor protein gene.J Biol Chem.1998;273(46):30366-30371.
- Karimi F, Haghighi AB, Petramfar P. Low Levels of Triiodothyronine in Patients with Alzheimer’s Disease.Iran J Med Sci. 2011;36(4):322-323.
- Currier DG, Polk RC, Reeves RH. A Sonic hedgehog (Shh) response deficit in trisomic cells may be a common denominator for multiple features of Down syndrome.Prog Brain Res. 2012;197:223-236.
- Gutierrez-Castellanos N, Winkelman BHJ, Tolosa-Rodriguez L, et al. Size does not always matter: Ts65Dn Down syndrome mice show cerebellum-dependent motor learning deficits that cannot be rescued by postnatal SAG treatment.J Neurosci. 2013;33(39):15408-15413.
- Das I, Park J-M, Shin JH, et al. Hedgehog agonist therapy corrects structural and cognitive deficits in a Down syndrome mouse model.Sci Transl Med. 2013;5(201):201ra120.
- Desouza LA, Sathanoori M, Kapoor R, et al. Thyroid hormone regulates the expression of the sonic hedgehog signaling pathway in the embryonic and adult mammalian brain.Endocrinol. 2011;152(5):1989-2000.
- Letourneau A, Santoni FA, Bonilla X, et al. Domains of genome-wide gene expression dysregulation in Down’s syndrome.Nature. 2014;508:345-350
- Mendioroz M, Do C, Jiang X, et al.Trans effects of chromosome aneuploidies on DNA methylation patterns in human Down syndrome and mouse models. Genome Biol. 2015;16:263.
- Suetake I, Shinozaki F, Miyagawa J, Takeshima H, Tajima S.DNMT3L stimulates the DNA methylation activity of Dnmt3a and Dnmt3b through a direct interaction. J Biol Chem 2004;279:27816-27823.
- Kyono Y,Sachs LM, Bilesimo P, et al. Developmental and thyroid hormone regulation of the DNA methyltransferase 3a gene in Xenopus tadpoles. Endocrinol. 2016;157(12):4961-4972.
- Wu Y, Koenig RJ. Gene regulation by thyroid hormone.Trends Endocrinol Metab. 2000;11:207-211.
- Ayers S, Switnicki MP, Angajala A, et al. Genome-wide binding patterns of thyroid hormone receptor beta. Sladek FM, ed.PLoS ONE. 2014;9(2):e81186.
- Blazek JD,Malik AM, Tischbein M, Arbones ML, Moore CS, Roper RJ. Abnormal mineralization of the Ts65Dn Down syndrome mouse appendicular skeleton begins during embryonic development in a Dyrk1a-independent manner. Mech Dev. 2015 May;136:133-42.
- Blazek JD, Gaddy A, Meyer R, et al. Disruption of bone development and homeostasis by trisomy in Ts65Dn Down syndrome mice.Bone. 2011;48(2):275-280.
- Bassett JH, Nordstrom K, Boyde A, et al. Thyroid status during skeletal development determines adult bone structure and mineralization.Mol Endocrinol. 2007;21:1893-1904.
- Waung JA, Bassett JHD, Williams GR. Thyroid hormone metabolism in skeletal development and adult bone maintenance.Trends Endocrinol Metab. 2012;23:155-162.
- Fernandez F, Edgin JO. Poor sleep as a precursor to cognitive decline in Down syndrome: A hypothesis.J Alzheimers Dis Parkinsonism. 2013;3(2):124.
- Chen CC, Spanò G, Edgin JO.The impact of sleep disruption on executive function in Down syndrome. Res Dev Disabil. 2013;34:2033-2039.
- Campos-Barros A, Musa A, Flechner A, et al.Evidence for circadian variations of thyroid hormone concentrations and type II 5′-iodothyronine deiodinase activity in the rat central nervous system. J Neurochem 1997;68:795-803.
- Murakami N, Hayafuji C, Takahashi K. Thyroid hormone maintains normal circadian rhythm of blood corticosterone levels in the rat by restoring the release and synthesis of ACTH after thyroidectomy.Acta Endocrinologica. 1984;107(4):519-524.
- Steiger A. Thyroid gland and sleep. Acta Med Austriaca.1999;26(4):132-133.
- Pagano G, Castello G. Oxidative stress and mitochondrial dysfunction in Down syndrome.Adv Exp Med Biol. 2012;724:291–299.
- Arbuzova S, Hutchin T, Cuckle H. Mitochondrial dysfunction and Down’s syndrome.BioEssays. 2002;24(8):681-684.
- Coskun PE, Wyrembak J, Derbereva O, et al. Systemic mitochondrial dysfunction and the etiology of Alzheimer’s disease and Down syndrome dementia.J Alzheimers Dis. 2010;20(Suppl 2):S293-S310.
- Wrutniak-Cabello C, Casas F, Cabello G. Thyroid hormone action in mitochondria.J Mol Endocrinol 2001;26:67-77.
- Lombardi A, Lanni A, Moreno M, et al. Effect of 3,5-di-iodo-L-thyronine on the mitochondrial energy-transduction apparatus.Biochem J. 1998;330(Pt 1):521-526.
- Lebon V, Dufour S, Petersen KF, et al. Effect of triiodothyronine on mitochondrial energy coupling in human skeletal muscle.J Clin Invest. 2001;108(5):733-737.
- Goglia F, Silvestri E, Lanni Thyroid hormones and mitochondria. Biosci Rep. 2002;22:17-32.
- Chopra IJ, Sack J, Fisher DA. Circulating 3,3′, 5′-triiodothyronine (reverse T3) in the human newborn. J Clin Invest. 1975;55(6):1137-1141.
- [1]Williams FL, Simpson J, Delahunty C, et al. Developmental trends in cord and postpartum serum thyroid hormones in preterm infants.J Clin Endocrinol Metab. 2004;89:5314-5320.
- Brown R. Disoders of the thyroid gland in infancy, childhood, and adolescence. Thyroid Disease Manager. http://www.thyroidmanager.org/chapter/disorders-of-the-thyroid-gland-in-infancy-childhood-and-adolescence/. Updated March 21, 2012. Accessed January 12, 2016.
Erica Peirson, ND, is a naturopathic physician and the mother of a 7-year-old son with Mosaic Down Syndrome who experienced undiagnosed hypothyroidism as an infant. She is dedicated to helping other children avoid this potentially devastating start in life. She sees patients privately at the Peirson Center for Children, and is the executive director of Down Syndrome OPTIONs. She writes and lectures whenever possible to help spread awareness to parents and physicians that the symptoms of Down syndrome are treatable through proper treatment and detection of hypothyroidism, among other things. Her knowledge gained working with these children has aided her in helping other children with special needs, including those with autism.