Mycotoxins & the Brain
Lauren Tessier, ND
If you’ve worked with people suffering from mold and mycotoxin exposure, you are aware of the vast array of symptoms they report. It is not uncommon for people to experience a litany of cognitive symptoms, including, but not limited to, brain fog, confusion, inattention, disorientation, and difficulties with memory, problem solving, following conversation, reaction time, and learning. Additional neurocognitive effects can manifest as mood complaints, such as depression, anxiety, obsessive-compulsive disorder (OCD)-like behavior, and social withdrawal. Meanwhile, strictly neurological complaints vary wildly and may involve reports of sensations of dizziness, internal vibration, numbness (which may be non-dermatomal), tingling, ataxia, vertigo, sharp pain, photophobia, changes in smell and taste, and tinnitus. Symptoms such as atypical seizure presentations, syncope, tremor, tics, depersonalization, and derealization may also occur, but to a lesser extent.
Many of the aforementioned neurocognitive complaints have been demonstrated in various studies of mold-exposed cohorts.1-7 A 2003 study found that 70 of 100 patients exposed to mold in their homes exhibited neurological symptoms that included ataxia, dizziness, and neurocognitive complaints.8 Moreover, assessment of their autonomic nervous system, via heart rate variability, demonstrated abnormalities in all who were tested. Neurocognitive assessments of a portion of study participants (limited due to cost) revealed deficits in problem solving, executive functioning, judgment, hand/eye coordination, and memory. Moreover, SPECT scans demonstrated a neurotoxic pattern in approximately 86% of a subgroup that clinically demonstrated imbalance.8 Another 2003 study, examining 182 mold-exposed patients, found hypoactivation of the frontal cortex, as demonstrated on quantitative electroencephalogram.9 These findings, in addition to the degree of exposure, correlated significantly to abnormal cognitive testing outcomes. It is obvious that the aforementioned neurological complaints are shared with many other serious conditions; therefore, it is imperative that all pertinent differential diagnoses are considered and ruled out. However, it should be noted that a proper work-up of mold illness should be included, as one should not underestimate the profound neurological impact of mold exposure.
Unfortunately, the nervous system stands to suffer grave ill effects following mold and mycotoxin exposure. The reason for this is multifactorial. Firstly, the brain is largely made of fatty tissue, and many mycotoxins are small and lipophilic in nature, thus able to pass through lipid-rich cell membranes with ease. Many lipophilic mycotoxins, such as enniatin B, beauvercin, and aflatoxin, have been found in high concentrations in tissues and organs with high fat content, such as liver and adipose tissue.10-12 Of course, the fatty tissue of the nervous system is no exception; such lipophilic mycotoxins do not spare the brain. In mice, in-vivo systemic administration of beauvercin and enniatin resulted in their deposition in brain tissue, demonstrating their ability to cross the blood-brain barrier (BBB).13
The lipophilic nature of mycotoxins presents additional concerns when one considers the direct communication between the olfactory bulb of the nose and the brain.14 In a 2006 mouse study, intranasal exposure to satratoxin G, the mycotoxin produced by the “infamous” black mold, Stachybotrys chartarum, resulted in apoptosis of olfactory sensory nerves.15Another mouse study delivered a similar apoptotic finding, in conjunction with bilateral atrophy of the olfactory nerve layer of the olfactory bulb of the brain.16
The high lipid content of the brain also places it at increased risk for lipid peroxidation, which is potentially made worse by the brain’s great demand for energy and oxygen. Oxidative damage, including lipid peroxidation, happens to be one of the most widely understood mechanisms of action of damage imparted by mycotoxins.17-36
Mycotoxins and the BBB
Understanding the impact of mycotoxins on the brain requires a quick refresher on astrocytes. These glial cells are an important part of the nervous system, as they are integral to the proper maintenance of the BBB.37 Astrocyte “endfeet” interact with the endothelial cells of the BBB to help direct production of various enzymes, development of tight junctions,38,39 and alteration of BBB permeability to various nutrients.40,41
There is direct evidence supporting mycotoxin-induced damage to astrocytes. A 2005 study of rat astrocytes demonstrated that sub-cytotoxic levels of ochratoxin A exposure damage the cytoskeleton of astrocytes, in conjunction with an upregulation of genes responsible for a neuroinflammatory response.42 Meanwhile, T-2 toxin, a Fusarium-generated trichothecene, has been shown to be cytotoxic to cultured human astrocytes at low concentrations and with relatively short exposure times.43 When astrocytes are injured, there is a disruption of ion homeostasis, specifically an intercellular calcium wave that propagates to endothelial cells, resulting in abnormalities of BBB permeability and transport.44 Direct evidence of such disruption has been demonstrated with human astrocyte exposure to ochratoxin A, which resulted in an increase in cytosolic calcium and apoptosis.45 It is without coincidence, then, that research has demonstrated mycotoxin-induced alterations of the BBB.46,47 An in-vivo mouse study revealed alterations of BBB permeability as a result of percutaneous exposures of brain and spleen tissue to T-2 toxin.48 Such T-2-induced alterations can impact permeability of amino acids,49 thereby shifting amino acid-dependent metabolism, such as the synthesis of neurotransmitters. However, mycotoxins/BBB abnormalities are not limited to alterations of permeability. Numerous mycotoxins have been shown to cross the BBB, such as aflatoxins,50-51 ochratoxin A,52 fumonisin B1,53 tricoherences,47,54-59 beauvericin,13 and enniatins,13,60 with some resulting brain deposition.
Mycotoxin Damage to Brain Regions
Research has also demonstrated that different mycotoxins may be toxic to various parts of the brain. For instance, intragastric administration of ochratoxin A has been shown in rats to result in brain deposition of the mycotoxin, leading to damage to the hippocampus, ventral mesencephalon, striatum, and cerebellum.52 Additional research in rats confirmed severe focal ochratoxin A-induced neurotoxicity of the hippocampus, as compared to other brain regions.61 Meanwhile, in mice, intraperitoneal injections of ochratoxin A resulted in marked damage to the midbrain, caudate putamen, and hippocampus.17 In a rat study, subchronic exposure of ochratoxin A caused a downregulation of NDMA receptors,62 which are crucial for learning and memory.63 An in-vivo study of the mouse subventricular zone, known for its neurogenic potential, demonstrated drastic changes when the tissue was exposed to ochratoxin A.64 Of note was a significant decrease in cell viability, cellular proliferation, differentiation, astrocyte counts, and neurogenesis.
A 2000 rat study revealed reduced excitability of the neocortex following fumonisin exposure, which manifested as disturbances in information processing.65 Fumonisin exposure has also been implicated in the demyelination of the forebrain in rats.66 In a 2017 in-vivo study, the pituitary gland was implicated as a target organ for T-2 toxin injury.67 While specific brain regions may suffer damage at the hands of mycotoxins, general tissue injury has also been widely documented. Fumonisin B1 is a known disruptor of myelination in both in-vivo68 and in-vitro rat studies,69 and has been shown to inhibit axonal growth in cultured hippocampal neurons.70 Gliotoxin has been demonstrated to be neurotoxic in numerous human in-vitro studies.71-73
Mycotoxin Damage to Brain Metabolism
Mycotoxins can also cause shifts in brain metabolism. For instance, ingestion of ochratoxin A-contaminated feed resulted in alterations of amino acid metabolism in the brains of rats.61 This mycotoxin has also been shown to interfere with the activity of phenylalanine hydroxylase,74 which is an integral enzyme in the formation of tyrosine and also a precursor of L-dopa and other downstream catecholamines. Mouse studies have revealed such a depletion of striatal dopamine,17,75 while in-vivo chicken studies have demonstrated dopamine depletion via exposure to deoxynivalenol,76 and serotonin and noradrenaline depletion as a result of ochratoxin A exposure.75 Fumonisin B1 has been shown to alter rat neurotransmitter metabolism in various brain regions of rats77 and to alter neurotransmitter concentrations in mice.78 In female rat models, acute systemic administration of T-2 toxin resulted in decreased brain stem and cerebellar serotonin, while chronic exposure resulted in elevated levels of serotonin in the cerebellum.79 Meanwhile, studies of T-2 exposed male rats showed elevated levels of monoamines80 and serotonin.80-82 In another in-vivo study, exposure to T-2 in male rats resulted in an increase in serotonin globally throughout the brain, as well as regional increases and decreases in norepinephrine.83 Of note, the dose used in this study was 0.1 mg/kg body weight, which represents only 2% of the LD50 value for T-2.
Closing Comments
While much of the research regarding mycotoxin impact on the brain comes from animal studies, it is very important that we pay attention to these findings. Many clients I see have been given a clean bill of health by their neurologist and primary care physician, but they continue to suffer. It is in these clients that mold and mycotoxin exposure should be considered. Clearly, it is typically the last thing to be ruled out, but that does not make the work-up unimportant. Many clients with proper support will see drastic improvements in their neurocognitive complaints – some in a few short weeks, whereas in others it may take 1 to 2 years.
When working with neurologically impacted mold-exposed clients, please be very mindful of the diagnosis codes you employ. In the world of preexisting conditions, one needs to be particularly cautious to avoid incorrect or overzealous diagnoses. For instance, a patient who is acutely mold exposed, demonstrating cognitive deficits and difficulties with short-term memory, may clinically present as an “inhalational Alzheimer’s” case.84 In 6 short months, they may have completely recovered, yet the poorly chosen diagnosis of “Alzheimer’s” may continue to impact their life, especially in the realm of disability insurance or life insurance. Thus, please be sure to only code what is truly diagnosable, understanding that your choices can impact the patient’s future for years to come.
References:
- Anyanwu E, Campbell AW, High W. Brainstem auditory evoked response in adolescents with acoustic mycotic neuroma due to environmental exposure to toxic molds. Int J Adolesc Med Health. 2002;14(1):67-76.
- Kilburn KH. Inhalation of molds and mycotoxins. Eur J Oncol. 2002;7:197-202.
- Gray MR, Thrasher JD, Crago R, et al. Mixed mold mycotoxicosis: immunological changes in humans following exposure in water-damaged buildings. Arch Environ Health. 2003;58(7):410-420.
- Auger P, Pepin P, Miller JD, et al. Health effects, pathology, epidemiology. In: Johanning E, ed. Bioaerosols, Fungi and Mycotoxins: Health Effects, Assessment, Prevention and Control. Albany, NY: Fungal Research Group Foundation; 2001.
- Anyanwu EC, Campbell AW, Vojdani A. Neurophysiological effects of chronic indoor environmental toxic mold exposure on children. ScientificWorldJournal. 2003;3:281-290.
- Gordon WA, Johanning E, Haddad L. Cognitive impairment associated with exposure to toxigenic fungi, health effects. In: Johanning E, ed. Bioaerosols, Fungi and Mycotoxins: Health Effects, Assessment, Prevention and Control. Albany, NY: Eastern New York Occupational and Environmental Health Center; 1999.
- Baldo JV, Ahmad L, Ruff R. Neuropsychological performance of patients following mold exposure. Appl Neuropsychol. 2002;9(4):193-202.
- Rea WJ, Didriksen N, Simon TR, et al. Effects of toxic exposure to molds and mycotoxins in building-related illnesses. Arch Environ Health. 2003;58(7):399-405.
- Crago BR, Gray MR, Nelson LA, et al. Psychological, neuropsychological, and electrocortical effects of mixed mold exposure. Arch Environ Health. 2003;58(8):452-463.
- Jonsson M, Jestoi M, Anthoni M, et al. Fusarium mycotoxin enniatin B: Cytotoxic effects and changes in gene expression profile. Toxicol In Vitro. 2016;34:309-320.
- Bbosa GS, Kitya D, Lubega A, et al. Review of the Biological and Health Effects of Aflatoxins on Body Organs and Body Systems. January 23, 2013. Available at: https://www.intechopen.com/books/aflatoxins-recent-advances-and-future-prospects/review-of-the-biological-and-health-effects-of-aflatoxins-on-body-organs-and-body-systems. Accessed March 5, 2020.
- Rodríguez-Carrasco Y, Heilos D, Richter L, et al. Mouse tissue distribution and persistence of the food-born fusariotoxins Enniatin B and Beauvericin. Toxicol Lett. 2016;247:35-44.
- Taevernier L, Bracke N, Veryser L, et al. Blood-brain barrier transport kinetics of the cyclic depsipeptide mycotoxins beauvericin and enniatins. Toxicol Lett. 2016;258:175-184.
- Dando SJ, Mackay-Sim A, Norton R, et al. Pathogens penetrating the central nervous system: infection pathways and the cellular and molecular mechanisms of invasion. Clin Microbiol Rev. 2014;27(4):691-726. Islam Z, Harkema JR, Pestka JJ. Satratoxin G from the black mold Stachybotrys chartarum evokes olfactory sensory neuron loss and inflammation in the murine nose and brain. Environ Health Perspect. 2006;114(7):1099-1107.
- Islam Z, Amuzie CJ, Harkema JR, Pestka JJ. Neurotoxicity and inflammation in the nasal airways of mice exposed to the macrocyclic trichothecene mycotoxin roridin a: kinetics and potentiation by bacterial lipopolysaccharide coexposure. Toxicol Sci. 2007;98(2):526-541.
- Sava V, Reunova O, Velasquez A, et al. Acute neurotoxic effects of the fungal metabolite ochratoxin-A. Neurotoxicology. 2006;27(1):82-92.
- Chaudhary M, Rao PVL. Brain oxidative stress after dermal and subcutaneous exposure of T-2 toxin in mice. Food Chem Toxicol. 2010;48(12):3436-3442.
- Sava V, Reunova O, Velasquez A, Sanchez-Ramos J. Can low level exposure to ochratoxin-A cause parkinsonism? J Neurol Sci. 2006;249(1):68-75.
- Sundstøl Eriksen G, Pettersson H, Lundh T. Comparative cytotoxicity of deoxynivalenol, nivalenol, their acetylated derivatives and de-epoxy metabolites. Food Chem Toxicol. 2004;42(4):619-624.
- Mézes M, Barta M, Nagy G. Comparative investigation on the effect of T-2 mycotoxin on lipid peroxidation and antioxidant status in different poultry species. Res Vet Sci. 1999;66(1):19-23.
- Mobio TA, Tavan E, Baudrimont I, et al. Comparative study of the toxic effects of fumonisin B1 in rat C6 glioma cells and p53-null mouse embryo fibroblasts. Toxicology. 2003;183(1-3):65-75.
- Abado-Becognee K, Mobio TA, Ennamany R, et al. Cytotoxicity of fumonisin B1: implication of lipid peroxidation and inhibition of protein and DNA syntheses. Arch Toxicol. 1998;72(4):233-236.
- Leal M, Shimada A, Ruı́z F, González de Mejı́a E. Effect of lycopene on lipid peroxidation and glutathione-dependent enzymes induced by T-2 toxin in vivo. Toxicol Lett. 1999;109(1-2):1-10.
- Stockmann-Juvala H, Mikkola J, Naarala J, et al. Fumonisin B1-induced toxicity and oxidative damage in U-118MG glioblastoma cells. Toxicology. 2004;202(3):173-183.
- Rahimtula AD, Béréziat JC, Bussacchini-Griot V, Bartsch H. Lipid peroxidation as a possible cause of ochratoxin a toxicity. Biochem Pharmacol. 1988;37(23):4469-4477.
- Rastogi R, Srivastava AK, Rastogi AK. Long term effect of aflatoxin B(1) on lipid peroxidation in rat liver and kidney: effect of picroliv and silymarin. Phytother Res. 2001;15(4):307-310.
- Doi K, Uetsuka K. Mechanisms of mycotoxin-induced neurotoxicity through oxidative stress-associated pathways. Int J Mol Sci. 2011;12(8):5213-5237.
- Ahmed N, Ram GC. Nuclear lipid peroxidation induced in rat liver by T-2 mycotoxin. Toxicon. 1986;24(9):947-949.
- Stockmann-Juvala H, Mikkola J, Naarala J, et al. Oxidative stress induced by fumonisin B1 in continuous human and rodent neural cell cultures. Free Radic Res. 2004;38(9):933-942.
- Kumar M, Dwivedi P, Sharma AK, et al. Apoptosis and lipid peroxidation in ochratoxin A- and citrinin-induced nephrotoxicity in rabbits. Toxicol Ind Health. 2014;30(1):90-98.
- Juan-García A, Juan C, Tolosa J, Ruiz MJ. Effects of deoxynivalenol, 3-acetyl-deoxynivalenol and 15-acetyl-deoxynivalenol on parameters associated with oxidative stress in HepG2 cells. Mycotoxin Res. 2019;35(2):197-205.
- da Silva EO, Gerez JR, Hohmann MSN, et al. Phytic Acid Decreases Oxidative Stress and Intestinal Lesions Induced by Fumonisin B₁ and Deoxynivalenol in Intestinal Explants of Pigs. Toxins (Basel). 2019 Jan 4;11(1). pii: E18. doi: 10.3390/toxins11010018.
- Xiao Y, Xu S, Zhao S, et al. Protective effects of selenium against zearalenone-induced apoptosis in chicken spleen lymphocyte via an endoplasmic reticulum stress signaling pathway. Cell Stress Chaperones. 2019;24(1):77-89.
- Müller LKF, Paiano D, Bottari NB, et al. Spray-dried porcine plasma added to diets contaminated with aflatoxins and fumonisins shows beneficial effects to piglet health. An Acad Bras Cienc. 2018;90(3):3115-3128.
- Mallebrera B, Maietti A, Tedeschi P, et al. Antioxidant capacity of trans-resveratrol dietary supplements alone or combined with the mycotoxin beauvericin. Food Chem Toxicol. 2017;105:315-318.
- Abbott NJ, Patabendige AA, Dolman DE, et al. Structure and function of the blood-brain barrier. Neurobiol Dis. 2010;37(1):13-25.
- Blackburn D, Sargsyan S, Monk PN, Shaw PJ. Astrocyte function and role in motor neuron disease: a future therapeutic target? Glia. 2009;57(12):1251-1264.
- Abbott NJ, Ronnback L, Hansson E. Astrocyte-endothelial interactions at the blood-brain barrier. Nat Rev Neurosci. 2006;7(1):41-53.
- Leybaert L. Neurobarrier coupling in the brain: A partner of neurovascular and neurometabolic coupling? J Cereb Blood Flow Metab. 2005;25(1):2-16.
- Benarroch EE. Astrocyte signaling and synaptic homeostasis: I: Membrane channels, transporters, and receptors in astrocytes. Neurology. 2016;87(3):324-330.
- Zurich MG, Lengacher S, Braissant O, et al. Unusual astrocyte reactivity caused by the food mycotoxin ochratoxin A in aggregating rat brain cell cultures. Neuroscience. 2005;134(3):771-782.
- Weidner M, Lenczyk M, Schwerdt G, et al. Neurotoxic potential and cellular uptake of T-2 toxin in human astrocytes in primary culture. Chem Res Toxicol. 2013;26(3):347-355.
- Paemeleire K, Leybaert L. ATP-dependent astrocyte-endothelial calcium signaling following mechanical damage to a single astrocyte in astrocyte-endothelial co-cultures. J Neurotrauma. 2000;17(4):345-358.
- Park S, Lim W, You S, Song G. Ochratoxin A exerts neurotoxicity in human astrocytes through mitochondria-dependent apoptosis and intracellular calcium overload. Toxicol Lett. 2019;313:42-49.
- Qureshi H, Hamid SS, Ali SS, et al. Cytotoxic effects of aflatoxin B1 on human brain microvascular endothelial cells of the blood-brain barrier. Med Mycol. 2015;53(4):409-416.
- Behrens M, Hüwel S, Galla HJ, Humpf HU. Blood-brain barrier effects of the Fusarium mycotoxins deoxynivalenol, 3 acetyldeoxynivalenol, and moniliformin and their transfer to the brain. PloS One. 2015;10(11):e0143640.
- Ravindran J, Agrawal M, Gupta N, Rao PV. Alteration of blood brain barrier permeability by T-2 toxin: Role of MMP-9 and inflammatory cytokines. Toxicology. 2011;280(1-2):44-52.
- Wang J, Fitzpatrick DW, Wilson JR. Effect of T-2 toxin on blood-brain barrier permeability monoamine oxidase activity and protein synthesis in rats. Food Chem Toxicol. 1998;36(11):955-961.
- Oyelami OA, Maxwell SM, Adelusola KA, et al. Aflatoxins in the autopsy brain tissue of children in Nigeria. Mycopathologia. 1995;132:35-38.
- Waggas AM, Rawi SM. Impact of 90-Day oral dosing with naturally occurring aflatoxin mixture on male sprague-dawley rats neurochemistry and behavioral pattern Middle-East. J Sci Res. 2013;14(2):228-238.
- Belmadani A, Tramu G, Betbeder AM, et al. Regional selectivity to ochratoxin A, distribution and cytotoxicity in rat brain. Arch Toxicol. 1998;72(10):656-662.
- Osuchowski MF, He Q, Sharma RP. Fumonisin B1 toxicity in the brain during coexisting lipopolysaccharide-related endotoxemia in BALB/c mice. Toxicol Sci. 2003;72:252-253.
- Weidner M, Hüwel S, Ebert F, et al. Influence of T-2 and HT-2 toxin on the blood-brain barrier in vitro: new experimental hints for neurotoxic effects. PLoS One. 2013;8(3):e60484.
- Prelusky DB, Hartin KE, Trenholm HL. Distribution of deoxynivalenol in cerebral spinal fluid following administration to swine and sheep. J Environ Sci Health B. 1990;25(3):395-413.
- Maresca M. From the gut to the brain: journey and pathophysiological effects of the food-associated trichothecene mycotoxin deoxynivalenol. Toxins (Basel). 2013;5(4):784-820.
- Pestka JJ, Islam Z, Amuzie CJ. Immunochemical assessment of deoxynivalenol tissue distribution following oral exposure in the mouse. Toxicol Lett. 2008;178(2):83-87.
- Foreman JH, Constable PD, Waggoner AL, et al. Neurologic abnormalities and cerebrospinal fluid changes in horses administered fumonisin B1 intravenously. J Vet Intern Med. 2004;18(2):223-230.
- Guo P, Liu A, Huang D, et al. Brain damage and neurological symptoms induced by T-2 toxin in rat brain. Toxicol Lett. 2018;286:96-107.
- Krug I, Behrens M, Esselen M, Humpf HU. Transport of enniatin B and enniatin B1 across the blood-brain barrier and hints for neurotoxic effects in cerebral cells. PLoS One. 2018;13(5):e0197406.
- Belmadani A, Tramu G, Betbeder AM, Creppy EE. Subchronic effects of ochratoxin A on young adult rat brain and partial prevention by aspartate, a sweetener. Hum Exp Toxicol. 1998;17(7):380-386.
- Delibas N, Altuntas I, Yonden Z, Ozcelik N. Ochratoxin A reduces NMDA receptor subunits 2A and 2B concentrations in rat hippocampus: partial protective effect of melatonin. Hum Exp Toxicol. 2003;22(6):335-339.
- Klann E. Cell permeable scavengers of superoxide prevent long-term potentiation in hippocampal area CA1. J Neurophysiol 1998;80(1):452-457.
- Ming GL, Song H. Adult neurogenesis in the mammalian brain: significant answers and significant questions. Neuron. 2011;70(4):687-702.
- Banczerowski-Pelyhe I, Világi I, Détri L, et al. In vivo and in vitro electrophysiological monitoring of rat neocortical activity after dietary fumonisin exposure. Mycopathologia. 2002;153(3):149-156.
- Kwon OS, Slikker W Jr, Davies DL. Biochemical and morphological effects of fumonisin B(1) on primary cultures of rat cerebrum. Neurotoxicol Teratol. 2000;22(4):565-572.
- Liu X, Huang D, Guo P, et al. PKA/CREB and NF-κB pathway regulates AKNA transcription: A novel insight into T-2 toxin-induced inflammation and GH deficiency in GH3 cells. Toxicology. 2017;392:81-95.
- Kwon OS, Schmued LC, Slikker W Jr. Fumonisin B1 in developing rats alters brain sphinganine levels andmyelination. Neurotoxicology. 1997;18(2):571-580.
- Monnet-Tschudi F, Zurich MG, Sorg O, et al. The naturally occurring food mycotoxin fumonisin B1 impairs myelin formation in aggregating brain cell culture. Neurotoxicology. 1999;20(1):41-48.
- Harel R, Futerman AH. Inhibition of sphingolipid synthesis affects axonal outgrowth in cultured hippocampal neurons. J Biol Chem. 1993;268(19):14476-14481.
- Speth C, Rambach G, Lass-Flörl C, et al. Culture supernatants of patient-derived Aspergillus isolates have toxic and lytic activity towards neurons and glial cells. FEMS Immunol Med Microbiol. 2000;29(4):303-313.
- Axelsson V, Holback S, Sjogren M, et al. Gliotoxin induces caspase-dependent neurite degeneration and calpain-mediated general cytotoxicity in differentiated human neuroblastoma SH-SY5Y cells. Biochem Biophys Res Commun. 2006;345(3):1068-1074.
- Speth C, Kupfahl C, Pfaller K, et al. Gliotoxin as putative virulence factor and immunotherapeutic target in a cell culture model of cerebral aspergillosis. Mol Immunol. 2011;48(15-16):2122–2129.
- Creppy EE, Chakor K, Fisher MJ, Dirheimer G. The myocotoxin ochratoxin A is a substrate for phenylalanine hydroxylase in isolated rat hepatocytes and in vivo. Arch Toxicol. 1990;64(4):279-284.
- Tamaru M, Hirata Y, Matsutani T. Neurochemical effects of prenatal treatment with ochratoxin A on fetal and adult mouse brain. Neurochem Res. 1988;13:1139-1147.
- Wang X, Tang J, Geng F, et al. Effects of deoxynivalenol exposure on cerebral lipid peroxidation, neurotransmitter and calcium homeostasis of chicks in vivo. Toxicon. 2018;150:60-65.
- Porter JK, Voss KA, Chamberlain WJ, et al. Neurotransmitters in rats fed fumonisin B1. Proc Soc Exp Biol Med. 1993;202(3):360-364.
- Tsunoda M, Dugyala RR, Sharma RP. Fumonisin B1-induced increases in neurotransmitter metabolite levels in different brain regions of BALB/c mice. Comp Biochem Physiol C Pharmacol Toxicol Endocrinol. 1998;120(3):457-465.
- Weekley LB, O’Rear CE, Kimbrough TD, Llewellyn GC. Acute and chronic effects of the trichothecene mycotoxin T-2 on rat brain regional concentrations of serotonin, tryptophan, and tyrosine. Vet Hum Toxicol. 1989;31(3):221-224.
- Wang J, Fitzpatrick DW, Wilson JR. Effect of dietary T-2 toxin on biogenic monoamines in discrete areas of the rat-brain. Food Chem Toxicol. 1993;31(3):191-197.
- Sheng K, Lu X, Yue J, et al. Role of neurotransmitters 5-hydroxytryptamine and substance P in anorexia induction following oral exposure to the trichothecene T-2 toxin. Food Chem Toxicol. 2019;123:1-8.
- Boyd KE, Fitzpatrick DW, Wilson JR, Wilson LM. Effect of T-2 toxin on brain biogenic monoamines in rats and chickens. Can J Vet Res. 1988;52(2):181-185.
- Wang J, Fitzpatrick DW, Wilson JR. Effects of the trichothecene mycotoxin T-2 toxin on neurotransmitters and metabolites in discrete areas of the rat brain. Food Chem Toxicol. 1998;36(11):947-953.
- Bredesen DE. Inhalational Alzheimer’s disease: an unrecognized – and treatable – epidemic. Aging (Albany NY). 2016;8(2):304-313.
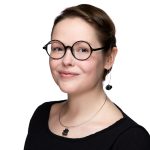
Lauren Tessier, ND, is a licensed naturopathic physician specializing in mold-related illness. She is a nationally known speaker and is the vice president of the International Society for Environmentally Acquired Illness (ISEAI) – a non-profit dedicated to educating physicians about the diagnosis and treatment of environmentally acquired illness. Dr Tessier’s practice, “Life After Mold,” in Waterbury, VT, draws clients from all around the world who suffer from chronic complex illness as a result of environmental exposure and chronic infections. Dr Tessier’s e-booklet, Mold Prevention: 101, has been widely circulated and its suggestions implemented by many worldwide.