Mold & Pediatrics
Tolle Causam
Lauren Tessier, ND
Air quality, as a part of the physical environment, is widely known as a determinant of health and, as such, has been widely studied. Everything from pollen or dust to volatile organic compounds (VOCs) has been examined in the medical literature. The harmful effects of mold have also been examined and confirmed, yet its acceptance seems to suffer bias.1
For something that supposedly does not have a deleterious impact, let alone on children, it is certainly curious that the danger of mold exposure is included as a point of concern in the investigation of sudden infant death syndrome (SIDS) cases. The Centers for Disease Control and Prevention (CDC) specifically asks about mold growth, standing water, and dampness in its investigation report form included in the 1996 document “Guidelines for Death Scene Investigation of Sudden, Unexplained Infant Deaths.”2 This alone speaks volumes. Fortunately, there is more to the discussion than mere speculation based on bureaucratic documents. Although the evidence 30 years ago was sparse, the field of study has grown significantly, and we have many outstanding researchers to thank for working hard to keep our children safe by bringing these hidden dangers to light.
One of the first documented case reports of human illness caused by airborne mycotoxin exposure occurred in the United States in the 1980s. The report discussed the symptoms experienced by a family, including 2 teens, that was exposed to the mycotoxin-producing black mold, Stachybotrys chartarum. Various complaints, including flu-like symptoms, diarrhea, sore throat, headache, hair loss, malaise, fatigue, and dermatitis, among others, were reported.3
Unfortunately, both the case report and its implications received little attention. Fast-forward to the early 1990s, when mold became headline news as numerous cases of acute pediatric pulmonary hemorrhage and hemosiderosis were reported in Cleveland, OH. These cases, investigated by the CDC, seemed to share a common factor: these infants were all exposed to a moldy home environment.4,5
A follow-up case-control study, which compared the aforementioned infants to non-exposed controls, was able to confirm the correlation of pulmonary hemorrhage and hemosiderosis to water-damaged homes. In fact, the case-control study found that water damage occurred within 1 month of illness onset in all cases, and in most cases was concomitant with the infants’ first episode. What is perhaps more upsetting is that there was no attempt to remediate any of the water damage in any of the affected children’s homes.6
There are various understandable reasons as to why remediation did not occur; perhaps it was as a result of socioeconomic status or poor building management. Nevertheless, lack of awareness is an altogether unacceptable reason. However, the unaware parents of mold-exposed children should not be looked poorly upon in these instances, especially when some authors of medical literature deny the deleterious effects of mold and mycotoxin exposure.
Asthma, Allergy, & Immune Disruption
As asthma and allergy occupy much of the pediatric mold and mycotoxin literature, there is little reason to debate a point so widely accepted. There are plenty of articles linking asthma, asthma-like symptoms, and atopic reactions in children to dampness and mold exposure.7-26
A key recurring finding coming out of the pediatric mold allergy literature has interesting implications for those who are exposed. There are a few studies that have demonstrated that children who are mold-exposed can become more sensitized to non-mold allergens. Although these children may not have positive IgE titers to specific molds, they have an increased IgE response to common allergens. The theory therein is that mold exposure somehow sensitizes children to become more atopic in nature to their environment.24
For example, a 2001 Finnish pediatric study examined the development of atopic complaints following exposure to a moldy and water-damaged school.27 Children who were exposed to an exceptionally water-damaged classroom reported a higher rate of atopic reactions coinciding with their school year. These children, when compared to controls, exhibited an increase in generalized allergic sensitivity to the 10 most common allergens, as demonstrated by antigen-specific IgE elevations. Perhaps the most interesting finding was that, among the atopic children exposed to mold, only 1 child demonstrated IgE positivity to fungi, specifically Aspergillus fumigatus.27
Thus, the key takeaway is that the most deleterious impacts of mold exposure might be non-IgE-mediated sensitivities, suggesting that there is more to mold exposure than just “mold allergy,” as so many like to claim. This priming of the immune system to become sensitive to other allergens demonstrates the underlying immune system disruption inherent to mold exposure.28 However, sensitization is not the only type of immune system dysregulation that results from mold exposure. A 2016 study showed that children exposed to water-damaged homes exhibited an increase in systemic inflammation, specifically serum C-reactive protein (CRP).29 Moreover, correlations were found between water damage in the main living areas of the home and elevations of the proinflammatory cytokines interleukin (IL)-6, IL-B-1, and tumor necrosis factor-alpha (TNFα) in whole-blood cultures. A 1998 study, examining the immune systems of mold-exposed children, showed that chronic mold exposure resulted not only in a reduction in the CD4/CD8 ratio, but also in an elevation of CD3+ T-lymphocytes expressing CD45RO and CD29 (high).30 Elevations remained in place at follow-up testing. Less specific immune system dysregulation has even been noted with food-borne mycotoxin exposure, as confirmed by a 2003 Gambian study that demonstrated that children exposed to dietary aflatoxin experienced a significant reduction in salivary IgA.31
Meanwhile, other studies have correlated a connection between mold exposure and infectious illness. A 2018 cross-sectional study of children living in 8 Chinese cities revealed a relationship between childhood pneumonia and various indicators of water-damaged buildings. Dampness indicators, such as mold-like odor, visible mold, water staining, condensation, and damp items, were recorded, and a direct correlation was found between the number of dampness indicators and pneumonia incidence.32 A 1999 Finnish study examined the correlation between mold and moisture problems in school buildings and the respiratory health of the children in attendance.33 The study found that mold-exposed children experienced a higher incidence of lower respiratory tract infections, prolonged cough, and an increase in emergency room visits and use of antibiotics, when compared to unexposed controls.33 A 2016 Canadian study found a correlation between reported mold levels and higher rates of persistent colds, especially among asthmatic children.34 In 2017, the same authors confirmed a correlation between exposure to Cladosporium spp and increased incidence of persistent colds and school absenteeism.35 A 2018 Chinese study was able to correlate an increased risk of childhood pneumonia with perinatal and postnatal exposure to window pane condensation, mold exposure, and mold odor.36 That same year, a Romanian study of school-aged children demonstrated a correlation between an increased rate of flu-like illness and mold and dampness in the home, especially findings of mold growth and water leakage during the previous 12 months and mold and dampness issues in the children’s bedrooms.37 Another Finnish study, this one from 2000, noted a higher prevalence of infections in children who were exposed to a moldy school; this finding was paired with an increase in physician visits as well as an increased use of antibiotics.38 This study was also the first of its kind to demonstrate a decrease in respiratory morbidity following proper renovation of the mold-exposed school. Unfortunately, this was too late for the aforementioned children of Cleveland, OH.
Physical Development
Thus far, many of the physical development issues we see in the medical literature center on food-borne exposures. However, one would be remiss to not make note of the deleterious effect of mycotoxins, no matter the route of exposure. Mycotoxins are present in all food chains worldwide, and to disregard such exposures would be foolish, especially when there is so much medical information on the topic. However, this discussion should also include vertical transmission from mother to child, as both breast milk exposure39-49 and in-utero exposure have been demonstrated. For example, various studies have demonstrated correlations between in-utero aflatoxin exposure and reduced height for age, and weight for age, in infants,50-56 as well as increased risk of neonatal jaundice.57
Other findings in children relate directly to the consumption of mycotoxin-contaminated foods. Decreased insulin-like growth factor (IGF)-1 and IGF binding protein-3 (IGFBP3) in infants and children have been associated with aflatoxin exposure, which may potentially account for reports of decreased weight and height.58-59 A similar pattern was found between dietary fumonisin exposure and a reduction in height for age in both infants and young children.60-61 Additional mycotoxins, such as zearalenone, have been correlated with a higher weight and height in girls with precocious puberty compared to controls.62
However, height and weight are not the only abnormal growth parameters that have been reported to result from mycotoxin exposure. Accounts of mycotoxin-induced precocious development have been reported from around the world. In the late 1970s, 272 young girls in Puerto Rico, ages 6 months to 8 years, experienced precocious puberty, and all of these children displayed increased blood concentrations of the anabolic mycotoxin, zearalenone.63-65 In a 1997 Turkish study, girls who demonstrated early breast bud development (thelarche) had levels of zearalenone that were 2-fold that of controls.66 Moreover, the girls who demonstrated precocious puberty showed a 2.8-fold level of the mycotoxin compared to controls. Meanwhile, a 2011 New Jersey study of 163 girls, ages 9-10, examined possible relationships between the girls’ development and urinary mycoestrogens.67 In 78.5% of cases analyzed, elevations of zearalenone were found in the urine, and these cases demonstrated shorter height and a lesser likelihood to have reached thelarche. The findings from the aforementioned studies are particularly interesting in light of the fact that animals studies have demonstrated zearalenone to have variable effects at different time-frames of development.68,69
Mental, Neurological, & Social Development
Damage inflicted by mold and mycotoxin exposure is not limited to precocious puberty or irregularities in height and weight. Mycotoxins are capable of adversely affecting the nervous system, via both food-borne and inhalational exposure. An example of such concerns was a 2006 case-control study that found an increased risk of neural tube defects as a result of maternal dietary exposure to fumonisin.70 The correlation was dose dependent, up until an abortifacient threshold. This link has been confirmed in numerous mouse models71-75 and is further supported by the increased prevalence of neural tube defects in regions where people consume foods rich in fumonisin.76–80
However, lasting neurological impacts extend beyond in-utero exposure. As discussed in previous articles, environmental mold and mycotoxin exposures can have a deleterious impact on the nervous system, and children, as humans, are no exception. Neurological complaints in children may manifest as attention deficit disorder (ADD)-like behavior, photophobia, dizziness, visual disturbances, tiredness/fatigue, confusion, headache, and learning difficulties, etc. A 2003 study showed that previously healthy children developed various symptoms as a result of living in homes with mold exposure.81 All of the children complained of fatigue, headache, dizziness, cough, and nosebleeds, and at least 50% of the children complained of nausea, shortness of breath without exertion, feelings of exhaustion, palpitations, photophobia, burning eyes, tinnitus, hearing loss, runny nose, poor appetite, weakness, and/or numbness and tingling of limbs. Symptoms of ear discharge, ataxia, and abdominal pains were limited to 40% of the study participants.81 This study examined neurological impacts as well, which amounted to abnormal findings in 70% of participants. Visually Evoked Potential (VEP) testing was performed, which revealed a bilateral prolongation of latencies, potentially indicative of optic nerve dysfunction. Additionally, Somatosensory Evoked Potentials (SSEP) testing demonstrated latency prolongation and slowing of conduction in those exposed to mold, as compared to none in controls; these findings were suggestive of neurological damage.81
A 2016 prospective cohort study, examining the neuropsychological development of children living in damp homes, demonstrated that exposure to such environments correlated with lower scores on the McCarthy Scales of Child Abilities, and the California Preschool Social Competency Scale.82 Another prospective case cohort study, conducted in 2009, revealed a positive correlation between prevalence of hyperactivity and inattention at age 10 and early exposure to a large array of fungal taxa within the home.83 A study of mold-exposed children on the autism spectrum demonstrated more neurobehavioral abnormalities, as compared to non-mold-exposed controls.84 The most prominent findings were balance issues, visual field abnormalities, and lengthened blink reflex latency. Also noted were neuropsychological abnormalities of peg placement, fingertip number writing, picture completion, and digit symbol substitution. Moreover, the mold-exposed children showed higher scores on the long-form Profile of Mood Status (POMS) compared to controls, indicative of negative and difficult emotional states.84 A profoundly concerning prospective birth cohort study examined the lasting impact of early postnatal mold exposure on the cognitive development of children. Findings revealed that prolonged exposure not only placed the child at a 3-times-higher risk of having a lower IQ score, but it also increased the severity of the IQ deficit.85
While some folks may argue that comparing food-borne exposure to inhalation exposure is like comparing apples to oranges, we need to be mindful that, similar to inhalation exposure, an oral route of exposure can have systemic effects. Perhaps one of the most upsetting demonstrations of this comes from a 1995 Nigerian pediatric postmortem study.86 Among 37 children who had died from various causes, aflatoxins were present in the cerebrums of 81% of cases; moreover, approximately 38% of cases showed more than 1 form of aflatoxin. Although these aflatoxins were found in the nervous system, the investigators concluded that that the aflatoxin exposure was likely through the diet.86
Closing Comment
While I make no claim to be an agent of sea change, I do hope that the reader will walk away from this piece understanding the inherent danger we place children in when we understate the hazards of mold and mycotoxin exposure. We owe it not only to our children, but also to the children who came before us; we are their voice, and it is our duty to protect them.
References:
- Chang C, Gershwin ME. The Myth of Mycotoxins and Mold Injury. Clin Rev Allergy Immunol. 2019;57(3):449-455.
- Centers for Disease Control and Prevention. Guidelines for Death Scene Investigation of Sudden, Unexplained Infant Deaths: Recommendations of the Interagency Panel on Sudden Infant Death Syndrome. June 21, 1996. CDC Web site. https://www.cdc.gov/mmwr/preview/mmwrhtml/00042657.htm. Accessed June 15, 2020.
- Croft WA, Jarvis BB, Yatawara CS. Airborne outbreak of trichothecene toxicosis. Atmos Environ. 1986;20(3):549-552.
- Centers for Disease Control and Prevention (CDC). Update: pulmonary hemorrhage/hemosiderosis among infants — Cleveland, Ohio, 1993-1996. MMWR Morb Mortal Wkly Rep. 1997;46(2)33-35.
- Centers for Disease Control and Prevention (CDC). Acute pulmonary hemorrhage/hemosiderosis among infants — Cleveland, January 1993-November 1994. MMWR Morb Mortal Wkly Rep. 1994;43(48):881-883.
- Montaña E, Etzel RA, Allan T, et al. Environmental risk factors associated with pediatric idiopathic pulmonary hemorrhage and hemosiderosis in a Cleveland community. Pediatrics. 1997;99(1):E5.
- Singh B, Singh S, Asif AR, et al. Allergic aspergillosis and the antigens of Aspergillus fumigatus. Curr Protein Pept Sci. 2014;15(5):403-423.
- Malling HJ. Diagnosis of mold allergy. Clin Rev Allergy. 1992;10(3):213-236.
- Niemeijer NR, de Monchy JG. Age-dependency of sensitization to aeroallergens in asthmatics. Allergy. 1992;47(4 Pt 2):431-435.
- Platt SD, Martin CJ, Hunt SM, Lewis CW. Damp housing, mold growth and symptomatic health state. Br Med J. 1989;298(6689):1673-1678.
- Andrae S, Axelson 0, Björkstén B, et al. Symptoms of bronchial hyperactivity and asthma in relation to environmental factors. Arch Dis Child. 1988;63(5):473-478.
- Strachan DP. Damp housing and childhood asthma: validation of reporting of symptoms. Br Med J. 1988;297(6658):1223-1226. [Published correction appears in BMJ 1988;297(6662):1500]
- Dales RE, Zwanenburg H, Burnett R, Franklin CA. Respiratory health effects of home dampness and mold among Canadian children. Am J Epidemiol. 1991;134(2):196-203.
- Koivikko A, Viander M, Lanner A. The use of the extended Phadebas RAST panel in the diagnosis of mould allergy in asthmatic children. Allergy. 1991;46(2):85-91.
- Jaakkola JK, Jaakkola N, Ruotsalainen R. Home dampness and mold as Determinants of respiratory symptoms and asthma in pre-school children. J Exp Anal Environ Epidemiol. 1993;3 Suppl 1:129-142.
- Seo S, Choung JT, Chen BT, et al. The level of submicron fungal fragments in homes with asthmatic children. Environ Res. 2014;131:71-76.
- Kirjavainen PV, Täubel M, Karvonen AM, et al. Microbial secondary metabolites in homes in association with moisture damage and asthma. Indoor Air. 2016;26(3):448-456.
- Brunekreef B, Dockery DW, Speizer FE, et al. Home dampness and respiratory morbidity in children. Am Rev Respir Dis. 1989;140(5):1363-1367.
- Simoni M, Lombardi E, Berti G, et al. Mould/dampness exposure at home is associated with respiratory disorders in Italian children and adolescents: the SIDRIA-2 Study. Occup Environ Med. 2005;62(9):616-622.
- Sinclair R, Russell C, Kray G, Vesper S. Asthma Risk Associated with Indoor Mold Contamination in Hispanic Communities in Eastern Coachella Valley, California. J Environ Public Health. 2018;2018:9350370.
- Fisk WJ, Chan WR, Johnson AL. Does dampness and mold in schools affect health? Results of a meta-analysis. Indoor Air. 2019;29(6):895-902.
- Thacher JD, Gruzieva O, Pershagen G, et al. Mold and dampness exposure and allergic outcomes from birth to adolescence: data from the BAMSE cohort. Allergy. 2017;72(6):967-974.
- Jones R, Recer GM, Hwang SA, Lin S. Association between indoor mold and asthma among children in Buffalo, New York. Indoor Air. 2011;21(2):156-164.
- Prester L. Indoor exposure to mould allergens. Arh Hig Rada Toksikol. 2011;62(4):371-380.
- Yazicioglu M, Asan A, Ones U, et al. Indoor airborne fungal spores and home characteristics in asthmatic children from Edirne region of Turkey. Allergol Immunopathol (Madr). 2004;32(4):197-203.
- Garrett MH, Rayment PR, Hooper MA, et al. Indoor airborne fungal spores, house dampness and associations with environmental factors and respiratory health in children. Clin Exp Allergy. 1998;28(4):459-467.
- Savilahti R, Uitti J, Roto P, et al. Increased prevalence of atopy among children exposed to mold in a school building. Allergy. 2001;56(2):175-179.
- Husman T. Health effects of indoor-air microorganisms. Scand J Work Environ Health. 1996;22(1):5-13.
- Mustonen K, Karvonen AM, Kirjavainen P, et al. Moisture damage in home associates with systemic inflammation in children. Indoor Air. 2016;26(3):439-447.
- Dales R, Dulberg C, Miller D, et al. Influence of residential fungal contamination on peripheral blood lymphocyte populations in children. Arch Environ Health. 1998;53(3):190-195.
- Turner PC, Moore SE, Hall AJ, et al. Modification of immune function through exposure to dietary aflatoxin in Gambian children. Environ Health Perspect. 2003;111(2):217-220.
- Zhuge Y, Qian H, Zheng X, et al. Residential risk factors for childhood pneumonia: A cross-sectional study in eight cities of China. Environ Int. 2018;116:83-91.
- Taskinen T, Hyvärinen A, Meklin T, et al. Asthma and respiratory infections in school children with special reference to moisture and mold problems in the school. Acta Paediatr. 1999;88(12):1373-1379.
- Polyzois D, Polyzoi E, Wells JA, Koulis T. Poor Indoor Air Quality, Mold Exposure, and Upper Respiratory Tract Infections–Are We Placing Our Children at Risk? J Environ Health. 2016;78(7):20-27.
- Polyzoi E, Polyzois D. Presence of Household Mold, Children’s Respiratory Health, and School Absenteeism: Cause for Concern. J Environ Health. 2017;79(7):28-35.
- Norbäck D, Lu C, Zhang Y, et al. Lifetime-ever pneumonia among pre-school children across China – Associations with pre-natal and post-natal early life environmental factors. Environ Res. 2018;167:418-427.
- Lu Y, Lin S, Lawrence WR, et al. Evidence from SINPHONIE project: Impact of home environmental exposures on respiratory health among school-age children in Romania. Sci Total Environ. 2018;621:75-84.
- Savilathi R, Uitti J, Laippala P, et al. Respiratory morbidity among children following renovation of a water damaged school. Arch Environ Health. 2000;55(6):405-410.
- Elaridi J, Bassil M, Kharma JA, et al. Analysis of Aflatoxin M1 in Breast Milk and Its Association with Nutritional and Socioeconomic Status of Lactating Mothers in Lebanon. J Food Prot. 2017;80(10):1737-1741.
- Diaz GJ, Sánchez MP. Determination of aflatoxin M1 in breast milk as a biomarker of maternal and infant exposure in Colombia. Food Addit Contam Part A Chem Anal Control Expo Risk Assess. 2015;32(7):1192-1198.
- Zarba A, Wild CP, Hall AJ, et al. Aflatoxin M1 in human breast milk from The Gambia, west Africa, quantified by combined monoclonal antibody immunoaffinity chromatography and HPLC. Carcinogenesis. 1992;13(5):891-894.
- Kılıç Altun S, Gürbüz S, Ayağ E. Aflatoxin M1 in human breast milk in southeastern Turkey. Mycotoxin Res. 2017;33(2):103-107.
- Magoha H, De Meulenaer B, Kimanya M, et al. Fumonisin B1 contamination in breast milk and its exposure in infants under 6 months of age in Rombo, Northern Tanzania. Food Chem Toxicol. 2014;74:112-116.
- Muñoz K, Blaszkewicz M, Campos V, Vega M, Degen GH. Exposure of infants to ochratoxin A with breast milk. Arch Toxicol. 2014;88(3):837-846.
- Memiş EY, Yalçın SS. Human milk mycotoxin contamination: smoking exposure and breastfeeding problems [published online ahead of print, 2019 Mar 8]. J Matern Fetal Neonatal Med. 2019;1-10.
- Dinleyici M, Aydemir O, Yildirim GK, et al. Human mature milk zearalenone and deoxynivalenol levels in Turkey. Neuro Endocrinol Lett. 2018;39(4):325-330.
- Braun D, Ezekiel CN, Abia WA, et al. Monitoring Early Life Mycotoxin Exposures via LC-MS/MS Breast Milk Analysis. Anal Chem. 2018;90(24):14569-14577.
- Vin K, Rivière G, Leconte S, et al. Dietary exposure to mycotoxins in the French infant total diet study [published online ahead of print, 2020 Mar 27]. Food Chem Toxicol. 2020;140:111301.
- Ortiz J, Jacxsens L, Astudillo G, et al. Multiple mycotoxin exposure of infants and young children via breastfeeding and complementary/weaning foods consumption in Ecuadorian highlands. Food Chem Toxicol. 2018;118:541-548.
- Abdulrazzaq YM, Osman N, Yousif ZM, Trad O. Morbidity in neonates of mothers who have ingested aflatoxins. Ann Trop Paediatr. 2004;24(2):145-151.
- De Vries HR, Maxwell SM, Hendrickse RG. Foetal and neonatal exposure to aflatoxins. Acta Paediatr Scand. 1989;78(3):373-378.
- Turner PC, Collinson AC, Cheung YB, et al. Aflatoxin exposure in utero causes growth faltering in Gambian infants. Int J Epidemiol. 2007;36(5):1119-1125.
- Gong Y, Hounsa A, Egal S, et al. Postweaning exposure to aflatoxin results in impaired child growth: A longitudinal study in Benin, West Africa. Environ Health Perspect. 2004;112(13):1334-1338.
- Gong YY, Cardwell K, Hounsa A, et al. Dietary aflatoxin exposure and impaired growth in young children from Benin and Togo: Cross-sectional study [published correction appears in BMJ 2002;325(7372):1089]. BMJ. 2002;325(7354):20-21.
- Gong YY, Egal S, Hounsa A, et al. Determinants of aflatoxin exposure in young children from Benin and Togo, West Africa: The critical role of weaning. Int J Epidemiol. 2003;32(4):556-562.
- Shouman BO, El Morsi D, Shabaan S, et al. Aflatoxin B1 level in relation to child’s feeding and growth. Indian J Pediatr. 2012;79(1):56-61.
- Sodeinde O, Chan MC, Maxwell SM, et al. Neonatal jaundice, aflatoxins and naphthols: report of a study in Ibadan, Nigeria. Ann Trop Paediatr. 1995;15(2):107-113.
- Castelino JM, Routledge MN, Wilson S, et al. Aflatoxin exposure is inversely associated with IGF1 and IGFBP3 levels in vitro and in Kenyan schoolchildren. Mol Nutr Food Res. 2015;59(3):574-581.
- Watson S, Moore SE, Darboe MK, et al. Impaired growth in rural Gambian infants exposed to aflatoxin: a prospective cohort study. BMC Public Health. 2018;18(1):1247.
- Shirima CP, Kimanya ME, Routledge MN, et al. A prospective study of growth and biomarkers of exposure to aflatoxin and fumonisin during early childhood in Tanzania. Environ Health Perspect. 2015;123(2):173-178.
- Kimanya ME, De Meulenaer B, Roberfroid D, et al. Fumonisin exposure through maize in complementary foods is inversely associated with linear growth of infants in Tanzania. Mol Nutr Food Res. 2010;54(11):1659-1667.
- Massart F, Meucci V, Saggese G, Soldani G. High growth rate of girls with precocious puberty exposed to estrogenic mycotoxins. J Pediatr. 2008;152(5):690-695.e1.
- Sáenz de Rodríguez CA. Environmental hormone contamination in Puerto Rico. N Engl J Med. 1984;310(26):1741-1742. Schoental R. Precocious sexual development in Puerto Rico and oestrogenic mycotoxins (zearalenone). Lancet. 1983;1(8323):537.
- Comas AP. Precocious sexual development in Puerto Rico. Lancet. 1982;1(8284):1299-1300.
- Asci A, Durmaz E, Erkekoglu P, et al. Urinary zearalenone levels in girls with premature thelarche and idiopathic central precocious puberty. Minerva Pediatra. 2014;66(6):571-578.
- Bandera EV, Chandran U, Buckley B, et al. Urinary mycoestrogens, body size and breast development in New Jersey girls. Sci Total Environ. 2011;409(24):5221-5227.
- Hilakivi-Clarke L, Onojafe I, Raygada M, et al. Prepubertal exposure to zearalenone or genistein reduces mammary tumorigenesis. Br J Cancer. 1999;80(11):1682-1688.
- Belli P, Bellaton C, Durand J, et al. Fetal and neonatal exposure to the mycotoxin zearalenone induces phenotypic alterations in adult rat mammary gland. Food Chem Toxicol. 2010;48(10):2818-2826.
- Missmer SA, Suarez L, Felkner M, et al. Exposure to fumonisins and the occurrence of neural tube defects along the Texas-Mexico border. Environ Health Perspect. 2006;114(2):237-241.
- Gelineau-van Waes J, Starr L, Maddox J, et al. Maternal fumonisin exposure and risk for neural tube defects: mechanisms in an in vivo mouse model. Birth Defects Res A Clin Mol Teratol. 2005;73(7):487-497.
- Ohta K, Maekawa M, Katagiri R, et al. Genetic susceptibility in the neural tube defects induced by ochratoxin A in the genetic arhinencephaly mouse, Pdn/Pdn. Congenit Anom (Kyoto). 2006;46(3):144-148.
- Marasas WF, Riley RT, Hendricks KA, et al. Fumonisins disrupt sphingolipid metabolism, folate transport, and neural tube development in embryo culture and in vivo: a potential risk factor for human neural tube defects among populations consuming fumonisin-contaminated maize. J Nutr. 2004;134(4):711-716.
- Katagiri R, Kurome M, Teshima Y, et al. Prevention of ochratoxin A-induced neural tube defects by folic acid in the genetic polydactyly/arhinencephaly mouse, Pdn/Pdn. Congenit Anom (Kyoto). 2007;47(3):90-96.
- Ueta E, Kodama M, Sumino Y, et al. Gender-dependent differences in the incidence of ochratoxin A-induced neural tube defects in the Pdn/Pdn mouse. Congenit Anom (Kyoto). 2010;50(1):29-39.
- Moore CA, Li S, Li Z, et al. Elevated rates of severe neural tube defects in a high prevalence area in northern China. Am J Med Genet. 1997;73(2):113-118.
- Ncayiyana DJ. Neural tube defects among rural blacks in a Transkei district. S Afr Med J. 1986;69(10):618-620.
- Venter PA, Christianson AL, Hutamo CM, et al. Congenital anomalies in rural black South African neonates—A silent epidemic. S Afr Med J. 1995;85(1):15-20.
- Kromberg JG, Jenkins T. Common birth defects in South African Blacks. S Afr Med J. 1982;62(17):599-602.
- Xiao KZ, Zhang ZY, Su YM, et al. Central nervous system congenital malformations, especially neural tube defects in 29 provinces, metropolitan cities and autonomous regions of China: Chinese Birth Defects Monitoring Program. Int J Epidemiol. 1990;19(4):978-982.
- Anyanwu EC, Campbell AW, Vojdani A. Neurophysiological effects of chronic indoor environmental toxic mold exposure on children. ScientificWorldJournal. 2003;3:281-290.
- Casas L, Torrent M, Zock JP, et al. Early life exposures to home dampness, pet ownership and farm animal contact and neuropsychological development in 4 year old children: a prospective birth cohort study. Int J Hyg Environ Health. 2013;216(6):690-697.
- Casas L, Karvonen AM, Kirjavainen PV, et al. Early life home microbiome and hyperactivity inattention in school-age children. Sci Rep. 2019;9(1):17355.
- Kilburn KH, Thrasher JD, Immers NB. Do terbutaline- and mold-associated impairments of the brain and lung relate to autism? Toxicol Ind Health. 2009;25(9-10):703-710.
- Jedrychowski W, Maugeri U, Perera F, et al. Cognitive function of 6-year old children exposed to mold-contaminated homes in early postnatal period. Prospective birth cohort study in Poland. Physiol Behav. 2011;104(5):989-995.
- Oyelami OA, Maxwell SM, Adelusola KA, et al. Aflatoxins in the autopsy brain tissue of children in Nigeria. Mycopathologia. 1995;132:35-38.
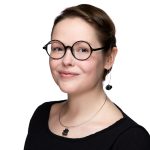
Lauren Tessier, ND, is a licensed naturopathic physician specializing in mold-related illness. She is a nationally known speaker and is the vice president of the International Society for Environmentally Acquired Illness (ISEAI) – a non-profit dedicated to educating physicians about the diagnosis and treatment of environmentally acquired illness. Dr Tessier’s practice, “Life After Mold,” in Waterbury, VT, draws clients from all around the world who suffer from chronic complex illness as a result of environmental exposure and chronic infections. Dr Tessier’s e-booklet, Mold Prevention: 101, has been widely circulated and its suggestions implemented by many worldwide.